Force work in si. Mechanical work
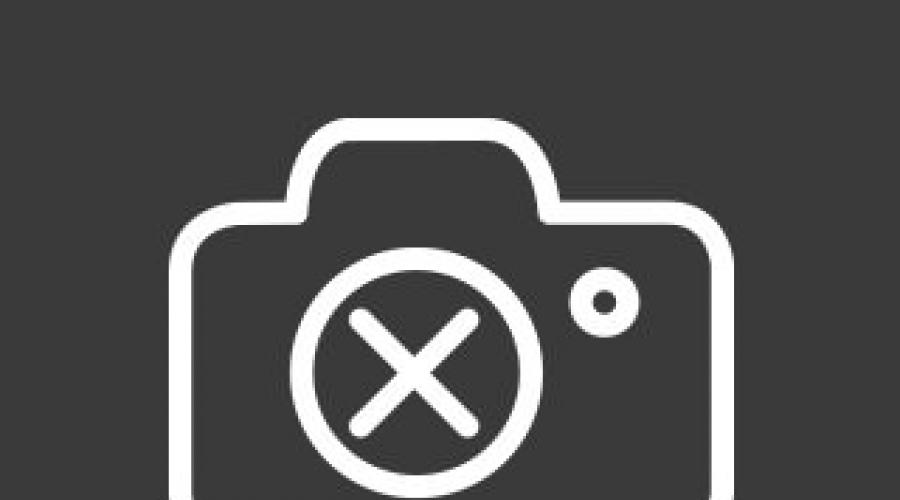
Read also
The horse pulls the cart with some force, let's designate it F traction. The grandfather, sitting on the cart, presses on her with some force. Let's denote it F pressure The cart moves in the direction of the horse's traction (to the right), but in the direction of the grandfather's pressure (down) the cart does not move. Therefore, in physics they say that F pulls does work on the cart, and F press does not work on the cart.
So, work of force on the body or mechanical work- a physical quantity, the modulus of which is equal to the product of the force by the path traversed by the body along the direction of action of these forces NS:
In honor of the English scientist D. Joule, the unit of mechanical work was named 1 joule(according to the formula, 1 J = 1 Nm).
If a certain force acts on the body in question, then some body acts on it. That's why work of force on the body and work of the body on the body are complete synonyms. However, the work of the first body on the second and the work of the second body on the first are partial synonyms, since the modules of these works are always equal, and their signs are always opposite. That is why the “±” sign is present in the formula. Let's discuss the signs of work in more detail.
The numerical values of force and path are always non-negative values. In contrast, mechanical work can have both positive and negative signs. If the direction of the force coincides with the direction of movement of the body, then force work is considered positive. If the direction of the force is opposite to the direction of movement of the body, work of force is considered negative(we take "-" from the "±" formula). If the direction of movement of the body is perpendicular to the direction of action of the force, then such a force does not perform work, that is, A = 0.
Consider three illustrations on three aspects of mechanical work.
Doing work by force can look different from the point of view of different observers. Consider an example: a girl is riding up in an elevator. Does she do mechanical work? A girl can only work on those bodies that she acts on by force. There is only one such body - an elevator car, as the girl presses on her floor with her weight. Now we need to find out if the cabin goes some way. Consider two options: with a stationary and a moving observer.
First have the observer boy sit on the ground. In relation to it, the elevator car moves up and travels a certain path. The girl's weight is directed in the opposite direction - down, therefore, the girl does negative mechanical work over the cabin: A virgins< 0. Вообразим, что мальчик-наблюдатель пересел внутрь кабины движущегося лифта. Как и ранее, вес девочки действует на пол кабины. Но теперь по отношению к такому наблюдателю кабина лифта не движется. Поэтому с точки зрения наблюдателя в кабине лифта девочка не совершает механическую работу: A dev = 0.
Efficiency shows the ratio of the useful work that is performed by a mechanism or device to that spent. Often, work expended is the amount of energy that the device consumes in order to get the job done.
You will need
- - automobile;
- - thermometer;
- - calculator.
Instructions
- To calculate the coefficient useful actions(Efficiency) divide the useful work Ap by the work expended by Az, and multiply the result by 100% (efficiency = Ap / Az ∙ 100%). Get the result as a percentage.
- When calculating the efficiency of a heat engine, consider the mechanical work performed by the mechanism as useful work. For the work expended, take the amount of heat released by the burnt fuel, which is the source of energy for the engine.
- Example. The average traction force of a car engine is 882 N. It consumes 7 kg of gasoline per 100 km of track. Determine the efficiency of its engine. Find useful work first. It is equal to the product of the force F by the distance S, overcome by the body under its influence Ap = F ∙ S. Determine the amount of heat that will be released when burning 7 kg of gasoline, this will be the spent work Az = Q = q ∙ m, where q is the specific heat of combustion of the fuel, for gasoline it is 42 ∙ 10 ^ 6 J / kg, and m is the mass of this fuel. The efficiency of the engine will be equal to the efficiency = (F ∙ S) / (q ∙ m) ∙ 100% = (882 ∙ 100000) / (42 ∙ 10 ^ 6 ∙ 7) ∙ 100% = 30%.
- In general, to find the efficiency, any heat engine (internal combustion engine, steam engine, turbine, etc.), where work is performed by gas, has a coefficient useful actions equal to the difference in the heat given by the heater Q1 and that obtained by the refrigerator Q2, find the difference in the heat of the heater and the refrigerator, and divide by the heat of the heater efficiency = (Q1-Q2) / Q1. Here, efficiency is measured in sub-multiples from 0 to 1, to convert the result to a percentage, multiply it by 100.
- To get the efficiency of an ideal heat engine (Carnot machine), find the ratio of the temperature difference between the heater T1 and the refrigerator T2 to the temperature of the heater efficiency = (T1-T2) / T1. This is the maximum possible efficiency for a specific type of heat engine with given temperatures of the heater and refrigerator.
- For an electric motor, find the work expended as the product of power and time. For example, if a crane electric motor with a power of 3.2 kW lifts a load weighing 800 kg to a height of 3.6 m in 10 s, then its efficiency is equal to the ratio of useful work Ap = m ∙ g ∙ h, where m is the mass of the load, g≈10 m / s² gravitational acceleration, h is the height to which the load was lifted, and the work expended Az = P ∙ t, where P is the engine power, t is its operating time. Get the formula for determining the efficiency = Ap / Az ∙ 100% = (m ∙ g ∙ h) / (R ∙ t) ∙ 100% =% = (800 ∙ 10 ∙ 3.6) / (3200 ∙ 10) ∙ 100% = 90%.
What is the formula for useful work?
Using this or that mechanism, we perform work that always exceeds that which is necessary to achieve the set goal. In accordance with this, a distinction is made between the complete or expended work of Az and the useful work of Ap. If, for example, our goal is to lift a load of mass m to a height H, then useful work is one that is conditioned only by overcoming the force of gravity acting on the load. With a uniform lifting of the load, when the force applied by us is equal to the gravity of the load, this work can be found as follows:
Ap = FH = mgH
What is work in physics definition of a formula. nn
Victor Chernobrovin
In physics, "mechanical work" is the work of some force (gravity, elasticity, friction, etc.) on a body, as a result of which the body moves. Sometimes you can find the expression "the body has done the work", which in principle means "the force acting on the body, has done the work."
Evgeny Makarov
Work is a physical quantity, numerically equal to the product of force and displacement in the direction of action of this force and caused by it.
Accordingly, the formula is A = F * s. If the displacement in the direction does not coincide with the direction of the action of the force, then the cosine of the angle appears.
Aysha allakulova
roman sparrows
Work is a process that requires the application of mental or physical efforts, which aims at obtaining a certain result. It is the work that, as a rule, determines the social status of a person. And it is, in fact, the main engine of progress in society. Work, as a phenomenon, is inherent only in living organisms and, first of all, in humans.
Mechanic
Mechanical work is a physical quantity that is a scalar quantitative measure of the action of a force or forces on a body or system, depending on the numerical value, the direction of the force (forces) and on the movement of the point (s), body or system.
Help me understand the formula !!
Syoma
in each specific case, we consider different useful energy, but usually this is work or heat that interested us (for example, the work of the gas to move the piston), and the energy expended is the energy that we betrayed to make our everything work (for example, the energy released when burning wood under a cylinder with a piston, inside of which is gas, which, expanding, did the work that we considered useful)
well, somehow it should be
Take a steam locomotive as an example.
For a steam locomotive to travel x km, you need to spend y tons of coal. When coal is burned, only Q1 of heat will be released, but not all heat is converted into useful work (according to the laws of thermodynamics, this is impossible). Useful work in this case is the movement of the locomotive.
Let the resistance force F act on the steam locomotive during movement (it arises due to friction in the mechanisms and due to other factors).
So, having passed x km, the steam locomotive will perform work Q2 = x * F
Thus,
Q1 - expended energy
Q2 - useful work
DeltaQ = (Q1 - Q2) is the energy spent on overcoming friction, on heating the surrounding air, etc.
Technical support
Efficiency - useful WORK to the expended.
For example, efficiency = 60%, heating takes 60 joules from the combustion of the substance. This is useful work.
We are interested in the spent, that is, how much of the total heat was released if 60 J.
Let's write it down.
Efficiency = Apol / Azatr
0.6 = 60 / Azatr
Azatr = 60 / 0.6 = 100J
As you can see, if a substance burns out at such an efficiency and 100 J (work expended) is released during combustion, then only 60% went into heating, that is, 60 J (useful work). The rest of the heat dissipated.
Prokhorov Anton
It must be understood in the literal sense: If we are talking about thermal energy, then we consider the energy expended that the fuel gives, and we consider the energy useful that they were able to use to achieve their goal, for example, what kind of energy a saucepan of water received.
Useful energy is always less expended!
Futynehf
Efficiency coefficient of efficiency degenerates as a percentage, characterizes the percentage that went to useful work from all spent. The simpler expended energy is the useful energy + the energy of heat loss in the system (if we are talking about heat, etc.) friction. heat with exhaust gases if referring to a car
Efficiency formula? is the work useful and complete?
Orbital constellation
Efficiency
Efficiency
(efficiency), characteristic of the efficiency of the system (device, machine) in relation to the transformation or transmission of energy; is determined by the ratio of useful energy used to the total amount of energy received by the system; usually denoted h = W total / Wcymmary.
In electric motors, efficiency is the ratio of the performed (useful) mechanical work to the electrical energy received from the source; in heat engines - the ratio of useful mechanical work to the consumed amount of heat; in electrical transformers - the ratio of electromagnetic energy received in the secondary winding to the energy consumed by the primary winding. To calculate efficiency, different types of energy and mechanical work are expressed in the same units based on the mechanical equivalent of heat, and other similar ratios. By virtue of its generality, the concept of efficiency makes it possible to compare and evaluate from a single point of view such different systems as nuclear reactors, electric generators and motors, thermal power plants, semiconductor devices, biological objects, etc.
http://ru.wikipedia.org/wiki/Power_Work
Field load is a term that is used in many areas of science and technology.
An "efficiency" parameter is often introduced as the ratio of the "weight" of the payload to the total "weight" of the system. In this case, "weight" can be measured both in kilograms / tons and bits (when transmitting packets over the network), or minutes / hours (when calculating the efficiency of processor time), or in other units.
http://ru.wikipedia.org/wiki/Useful_load
What is useful work and what is wasted work?
Vladimir Popov
Using this or that mechanism, we perform work that always exceeds that which is necessary to achieve the set goal. In accordance with this, a distinction is made between the complete or expended work of Az and the useful work of Ap. If, for example, our goal is to lift a load of mass w to a height H, then useful work is one that is conditioned only by overcoming the force of gravity acting on the load. With a uniform lifting of the load, when the force applied by us is equal to the gravity of the load, this work can be found as follows:
If we use a block or any other mechanism to lift a load, then, in addition to the gravity of the load, we also have to overcome the gravity of the parts of the mechanism, as well as the friction force acting in the mechanism. For example, using a movable block, we will have to do additional work to lift the block itself with a cable and to overcome the frictional force in the block axis. In addition, when we gain strength, we always lose along the way (more on this below), which also affects work. All this leads to the fact that the work we have spent turns out to be more useful:
Az> Ap.
Useful work is always only a fraction of the total work that a person does using the mechanism.
The physical quantity that shows what share of the useful work is from all the work expended is called the coefficient of efficiency of the mechanism.
Gorgeous
Efficiency (coefficient of performance) shows what proportion of the total work expended is useful work.
To find efficiency, you need to find the ratio of useful work to spent:
In physics, the concept of "work" has a different definition than that which is used in everyday life. In particular, the term "work" is used when a physical force causes an object to move. In general, if a powerful force makes an object move very far, then a lot of work is being done. And if the force is small or the object does not move very far, then only a little work. Strength can be calculated using the formula: Work = F × D × cosine (θ) where F = force (in Newtons), D = displacement (in meters), and θ = angle between the force vector and the direction of motion.
Steps
Part 1
Finding the value of work in one dimension-
Find the direction of the force vector and the direction of movement. To get started, it is important to first determine in which direction the object is moving, as well as where the force is being applied from. Keep in mind that objects do not always move in accordance with the force applied to them - for example, if you pull a small cart by the handle, then you are applying a diagonal force (if you are taller than the cart) to move it forward. In this section, however, we will deal with situations in which the force (effort) and movement of an object have same direction. For information on how to find a job when these items not have the same direction, read below.
- To make this process easy to understand, let's follow the example task. Let's say a toy carriage is pulled straight ahead by the train in front of it. In this case, the force vector and the direction of movement of the train indicate the same path - forward... In the next steps, we will use this information to help find the work done by the entity.
-
Find the offset of the object. The first variable D, or offset, that we need for the work formula is usually easy to find. Displacement is simply the distance the force caused the object to move from its original position. In educational tasks, this information, as a rule, is either given (known), or it can be derived (found) from other information in the task. In real life, all you have to do to find the displacement is to measure the distance the objects are moving.
- Note that distance units must be in meters in the formula to calculate the work.
- In our toy train example, let's say we find the work done by the train as it passes the track. If it starts at a certain point and stops at a place about 2 meters along the track, then we can use 2 meters for our "D" value in the formula.
-
Find the force applied to the object. Next, find the amount of force that is used to move the object. This is a measure of the "strength" of the force - the greater its value, the stronger it pushes the object and the faster it accelerates its course. If the magnitude of the force is not provided, it can be derived from the mass and the acceleration of displacement (provided that there are no other conflicting forces acting on it) using the formula F = M × A.
- Please note that the force units must be in Newtons to calculate the work formula.
- In our example, let's say we don't know the magnitude of the force. However, let's assume that know that the toy train has a mass of 0.5 kg and that the force makes it accelerate at a speed of 0.7 meters / second 2. In this case, we can find the value by multiplying M × A = 0.5 × 0.7 = 0.35 Newton.
-
Multiply Strength × Distance. Once you know the amount of force acting on your object and the distance it was moved, the rest is easy. Just multiply these two values by each other to get the work value.
- It's time to solve our example problem. With a force value of 0.35 Newton and a displacement value of 2 meters, our answer is a matter of simple multiplication: 0.35 × 2 = 0.7 Joules.
- You may have noticed that the formula given in the introduction has an extra part to the formula: cosine (θ). As discussed above, in this example, the force and direction of movement are applied in the same direction. This means that the angle between them is 0 o. Since cosine (0) = 1, we don't have to include it - we just multiply by 1.
-
Indicate the answer in Joules. In physics, the values of work (and several other quantities) are almost always given in a unit of measurement called the joule. One joule is defined as 1 Newton of force applied per meter, or in other words, 1 Newton × meter. This makes sense - since you are multiplying distance by force, it makes sense that the answer you get will have a unit of measurement equal to the unit of your force multiplied by your distance.
Part 2
Calculating work using angular force-
Find strength and displacement as usual. Above, we dealt with a problem in which an object moves in the same direction as the force that is applied to it. In fact, this is not always the case. In cases where the force and motion of an object are in two different directions, the difference between these two directions must also be factored into the equation to obtain an accurate result. First, find the amount of force and displacement of the object, as you usually do.
- Let's take a look at another example of a problem. In this case, suppose we are pulling the toy train forward as in the example problem above, but this time we are actually pulling upwards at a diagonal angle. In the next step, we will take this into account, but for now we will stick to the basics: the movement of the train and the magnitude of the force acting on it. For our purposes, let's say the force has the magnitude 10 Newton and that he drove the same 2 meters forward as before.
-
Find the angle between the force vector and the displacement. Unlike the examples above with a force that is in a different direction than the movement of the object, you need to find the difference between the two directions in terms of the angle between them. If this information is not provided to you, then you may need to measure the angle yourself or derive it from other information in the problem.
- In our example problem, suppose the force being applied is approximately 60 o above the horizontal plane. If the train is still moving straight ahead (that is, horizontally), then the angle between the vector of force and movement of the train will be equal to 60 o.
-
Multiply Force × Distance × Cosine (θ). Once you know the displacement of an object, the amount of force acting on it, and the angle between the force vector and its motion, the decision is almost as easy as without taking the angle into account. Just take the cosine of an angle (this may require a scientific calculator) and multiply it by force and displacement to find the answer to your problem in Joules.
- Let's solve an example of our problem. Using the calculator, we find that the cosine of 60 o is 1/2. Including this in the formula, we can solve the problem as follows: 10 Newtons × 2 meters × 1/2 = 10 Joules.
Part 3
Using the value of work-
Modify the formula to find distance, strength, or angle. The work formula above is not simply useful for finding work - it is also valuable for finding any variables in an equation when you already know the meaning of work. In these cases, simply highlight the variable you are looking for and solve the equation according to the basic rules of algebra.
- For example, suppose we know that our train is pulled with a force of 20 Newtons at a diagonal angle of more than 5 meters of track to do 86.6 Joules of work. However, we do not know the angle of the force vector. To find the angle, we simply select this variable and solve the equation like this: 86.6 = 20 × 5 × Cosine (θ) 86.6 / 100 = Cosine (θ) Arccos (0.866) = θ = 30 o
-
Divide by the time spent on the move to find the power. In physics, work is closely related to another type of measurement called "power". Power is simply a way of quantifying the amount of speed at which work is done on a particular system over a long period of time. Thus, to find the power, all you have to do is divide the work used to move the object by the time it takes to complete the move. Power measurements are indicated in units of W (which are equal to Joule / second).
- For example, for the example problem in the above step, suppose it took 12 seconds to move the train 5 meters. In this case, all you have to do is divide the work done to move it 5 meters (86.6 J) by 12 seconds to find the answer to calculate the power: 86.6 / 12 = " 7.22 Watt.
-
Use the formula TME i + W nc = TME f to find the mechanical energy in the system. The work can also be used to find the amount of energy contained in the system. In the above formula TME i = initial total mechanical energy in the TME system f = final the total mechanical energy in the system and W nc = work done in communication systems due to non-conservative forces. ... In this formula, if the force is applied in the direction of movement, then it is positive, and if it presses on (against) him, then it is negative. Note that both energy variables can be found by the formula (½) mv 2, where m = mass and V = volume.
- For example, for the example of the problem in two steps above, suppose that the train initially had a total mechanical energy of 100 J. Since the force in the problem pulls the train in the direction it has already passed, it is positive. In this case, the final energy of the train is TME i + W nc = 100 + 86.6 = 186.6 J.
- Note that non-conservative forces are forces whose power to affect the acceleration of an object depends on the path traveled by the object. Friction is a good example - an object pushed along a short, straight path will feel the effects of friction for a short time, while an object pushed along a long, winding path to the same final location will experience more friction overall.
- If you succeed in solving the problem, then smile and be glad for yourself!
- Practice solving as many problems as possible, this will ensure complete understanding.
- Keep practicing and try again if you fail the first time.
- Study the following points about work:
- The work done by force can be either positive or negative. (In this sense, the terms "positive or negative" carry their mathematical meaning, and their usual meaning).
- The work done is negative when the force is acting in the opposite direction to displacement.
- The work done is positive when the force is applied in the direction of travel.
-
The energy characteristics of motion are introduced on the basis of the concept of mechanical work or work of force.
Definition 1Work A performed by a constant force F → is a physical quantity equal to the product of the moduli of force and displacement multiplied by the cosine of the angle α located between the force vectors F → and displacement s →.
This definition is discussed in Figure 1. eighteen . 1 .
The work formula is written as,
A = F s cos α.
Work is a scalar. This makes it possible to be positive at (0 ° ≤ α< 90 °) , отрицательной при (90 ° < α ≤ 180 °) . Когда задается прямой угол α , тогда совершаемая сила равняется нулю. Единицы измерения работы по системе СИ - джоули (Д ж) .
A joule is equal to the work done by a force of 1 N to move 1 m in the direction of the force.
Picture 1 . eighteen . 1 . Work of force F →: A = F s cos α = F s s
With the projection F s → forces F → on the direction of displacement s → the force does not remain constant, and the calculation of work for small displacements Δ s i summed up and produced according to the formula:
A = ∑ ∆ A i = ∑ F s i ∆ s i.
This amount of work is calculated from the limit (Δ s i → 0), after which it turns into an integral.
The graphic representation of the work is determined from the area of the curvilinear figure located under the graph F s (x) of Figure 1. eighteen . 2.
Picture 1 . eighteen . 2. Graphical definition of work Δ A i = F s i Δ s i.
An example of a coordinate-dependent force is the elastic force of a spring that obeys Hooke's law. To stretch the spring, it is necessary to apply a force F →, the modulus of which is proportional to the elongation of the spring. This can be seen in Figure 1. eighteen . 3.
Picture 1 . eighteen . 3. Stretched spring. The direction of the external force F → coincides with the direction of displacement s →. F s = k x, where k denotes the stiffness of the spring.
F → y p p = - F →
The dependence of the modulus of the external force on the x coordinates can be plotted using a straight line.
Picture 1 . eighteen . 4 . Dependence of the modulus of the external force on the coordinate when the spring is stretched.
From the above figure, it is possible to find work on the external force of the right free end of the spring, using the area of the triangle. The formula will take the form
This formula is applicable to express the work done by an external force when a spring is compressed. Both cases show that the elastic force F → y p p is equal to the work of the external force F →, but with the opposite sign.
Definition 2
If several forces act on the body, then the formula for the total work will look like the sum of all the work done on it. When the body moves translationally, the points of application of the forces move in the same way, that is, the total work of all forces will be equal to the work of the resultant of the applied forces.
Picture 1 . eighteen . 5 . Mechanical work model.
Determination of power
Definition 3Power call the work of force performed per unit of time.
The record of the physical quantity of power, denoted by N, takes the form of the ratio of work A to the time interval t of the work being performed, that is:
Definition 4
The CI system uses watts (W t) as a unit of power, which is equal to the power of the force that performs work in 1 W in a time of 1 s.
If you notice an error in the text, please select it and press Ctrl + Enter
To be able to characterize the energy characteristics of motion, the concept of mechanical work was introduced. And it is to her in its various manifestations that the article is devoted. To understand, the topic is both easy and quite complex. The author sincerely tried to make it more comprehensible and understandable, and one can only hope that the goal has been achieved.
What is called mechanical work?
What is so called? If some force works on the body, and as a result of the action of this body, it moves, then this is called mechanical work. When approached from the point of view of scientific philosophy, several additional aspects can be distinguished here, but the article will cover the topic from the point of view of physics. Mechanical work is not difficult if you think carefully about the words written here. But the word "mechanical" is usually not written, and everything is shortened to the word "work". But not every job is mechanical. Here a man sits and thinks. Does it work? Mentally yes! But is it mechanical work? No. And if a person walks? If the body moves under the action of force, then this is mechanical work. It's simple. In other words, the force acting on the body does (mechanical) work. And one more thing: it is work that can characterize the result of the action of a certain force. So if a person walks, then certain forces (friction, gravity, etc.) perform mechanical work on the person, and as a result of their action, the person changes the point of his location, in other words, moves.
Work as a physical quantity is equal to the force that acts on the body, multiplied by the path that the body made under the influence of this force and in the direction indicated by it. We can say that mechanical work was done if 2 conditions were simultaneously met: the force acted on the body, and it moved in the direction of its action. But it did not take place or does not take place if the force acted and the body did not change its location in the coordinate system. Here are some small examples when mechanical work is not done:
- So a person can lean on a huge boulder in order to move it, but there is not enough strength. The force acts on the stone, but it does not move, and the work does not take place.
- The body moves in the coordinate system, and the force is equal to zero, or they are all compensated. This can be observed while moving by inertia.
- When the direction in which the body is moving is perpendicular to the action of the force. When the train moves along a horizontal line, the force of gravity does not do its work.
Depending on certain conditions, mechanical work is negative and positive. So, if the directions of both the forces and the movements of the body are the same, then positive work occurs. An example of positive work is the action of gravity on a falling drop of water. But if the force and direction of movement are opposite, then negative mechanical work occurs. An example of such a variant is a balloon rising up and the force of gravity, which does negative work. When the body succumbs to the influence of several forces, this work is called "the work of the resulting force."
Features of practical application (kinetic energy)
We pass from theory to practical part. Separately, we should talk about mechanical work and its use in physics. As many probably remembered, all the energy of the body is divided into kinetic and potential. When an object is in equilibrium and is not moving anywhere, its potential energy is equal to the total energy, and the kinetic energy is equal to zero. When movement begins, potential energy begins to decrease, kinetic energy begins to grow, but in total they equal the total energy of the object. For a material point, kinetic energy is defined as the work of the force that accelerated the point from zero to the value of H, and in formulaic form, the kinetics of a body is equal to ½ * M * H, where M is the mass. To find out the kinetic energy of an object that consists of many particles, it is necessary to find the sum of all the kinetic energy of the particles, and this will be the kinetic energy of the body.
Features of practical application (potential energy)
In the case when all forces acting on the body are conservative, and the potential energy is equal to the total, then the work is not done. This postulate is known as the law of conservation of mechanical energy. Mechanical energy in a closed system is constant over time. The conservation law is widely used to solve problems from classical mechanics.
Features of practical application (thermodynamics)
In thermodynamics, the work that a gas does during expansion is calculated from the integral of multiplying pressure by volume. This approach is applicable not only in cases where there is an exact function of volume, but also to all processes that can be displayed in the pressure / volume plane. Also, knowledge of mechanical work applies not only to gases, but to anything that can exert pressure.
Features of practical application in practice (theoretical mechanics)
In theoretical mechanics, all the properties and formulas described above are considered in more detail, in particular, these are projections. It also gives its own definition for various formulas of mechanical work (an example of the definition for the Rimmer integral): the limit to which the sum of all the forces of elementary work tends, when the fineness of the partition tends to zero, is called the work of the force along the curve. Probably difficult? But nothing, that's all with theoretical mechanics. And all the mechanical work, physics and other difficulties are over. Further there will be only examples and a conclusion.
Mechanical work units
Joules are used to measure work in SI, and the CGS uses erg:
- 1 J = 1 kgm² / s² = 1 Nm
- 1 erg = 1 gcm² / s² = 1 dyncm
- 1 erg = 10 −7 J
Examples of mechanical work
In order to finally understand such a concept as mechanical work, you should study several separate examples that will allow you to consider it from many, but not all sides:
- When a person lifts a stone with his hands, then mechanical work occurs with the help of the muscular strength of the hands;
- When a train travels on the rails, it is pulled by the traction force of the tractor (electric locomotive, diesel locomotive, etc.);
- If you take a gun and shoot from it, then thanks to the pressure force created by the powder gases, the job will be done: the bullet is moved along the barrel of the gun simultaneously with an increase in the speed of the bullet itself;
- Mechanical work is also present when the friction force acts on the body, forcing it to reduce the speed of its movement;
- The above example with balls, when they rise in the opposite direction relative to the direction of gravity, is also an example of mechanical work, but in addition to gravity, the Archimedes force also acts when everything that is lighter than air rises upward.
What is power?
Finally, I would like to touch on the topic of power. The work of force, which is performed in one unit of time, is called power. In fact, power is such a physical quantity that is a reflection of the ratio of work to a certain period of time during which this work was performed: M = P / B, where M is power, P is work, B is time. The SI unit of power is 1 W. A watt is equal to the power that does one joule of work in one second: 1 W = 1J / 1s.