Imaginary paradoxes of SRT. The Ehrenfest paradox
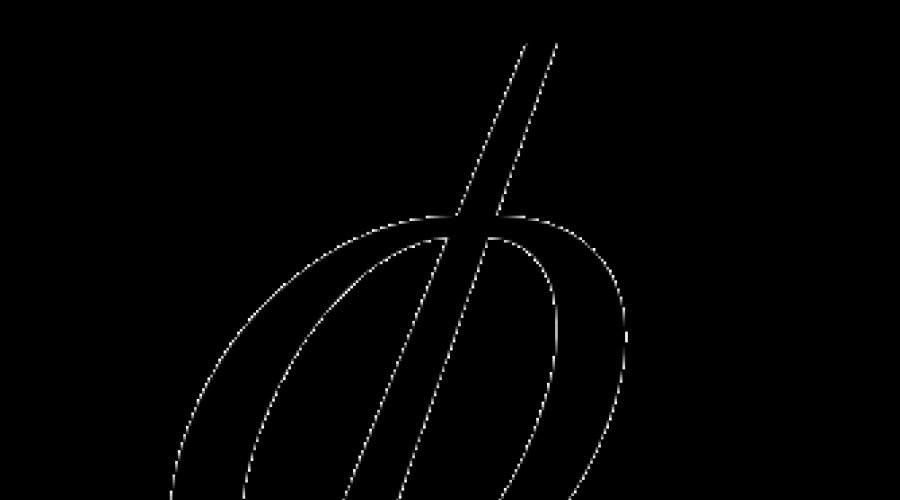
PARADOXES OF SPECIAL AND GENERAL THEORIES OF RELATIVITY
IN AND. Morenko
Abstract. This article is devoted to special relativity theory, Lorentz transformations and curvature of space-time. Isotropy and flatness of space have been experimentally proved but the theory (special and general relativity theories) demands different determination of space-time properties. Reasons of such disagreement are hidden in mathematical tools and methods used by the theories
The special theory of relativity is based on two, considered experimentally proven, facts - the finiteness of the speed of light and its constancy in various inertial reference frames (independence of the speed of light from its source). It is these conditions, according to the general opinion, do not allow the use of Galilean transformations in mechanics when passing from one inertial frame of reference to another. And, as a consequence, the relativistic principle of relativity based on Lorentz transformations is taken as the basis for the mathematical principles of describing the processes of motion. The evidence of these transformations seems so flawless that it would seem that there should not be any doubts about the validity of the conclusions arising from the application of the principle of Lorentz invariance in physical theory.
Indeed, in accordance with both postulates of the special theory of relativity (Einstein's relativistic principle of relativity and the principle of invariance of the speed of light in vacuum) for two inertial reference frames K and K’ , you can write:
In these equations, the components of the speed of light, provided that its propagation is straightforward:
Lorentz transformations preserve the invariance of the coordinate time during the transition from one local-inertial reference frame to another. However, these transformations were obtained in a very controversial way.
Indeed, Lorentz transformations are linear transformations of coordinates and time of two rectangular linear coordinate systems, one of which is stationary, and the second moves relative to the first with a speed V... To determine the correspondence of coordinates and times, a model is used to describe the motion of a single test photon (signal) from a single one at the zero moment of time for both systems of the origin O and to a common point for both systems M... And everything would be great if not for the fact that the trajectory of the test photon l f under given conditions cannot be straightforward at the same time in both coordinate systems K and K'Except in the obvious case where OO’ M - straight line. This statement follows from a comparison of the direction of the vector of rectilinear motion of the test photon in the system K with the direction of the vector of motion of the same photon in the system K’ ... Obviously, the components of the photon velocity in the system K obey the equation:
But in the system K’
these components are defined by the expression:
In this regard, the equation in the system K:
in system K’Can only be contrasted with the equation:
In these circumstances, the use of the linear transformation method to compare the coordinates and times of the systems K and K’ is, of course, an original, but hardly productive technique.
Thus, the special theory of relativity cannot be based on Lorentz invariance, but presupposes the freedom to choose the laboratory coordinate system, which is identical to the statement about the invariance of the mathematical form for determining the coordinate time in various locally inertial coordinate systems. The very same interpretation of SRT is a consequence of the neglect of the rules of mathematics (physicists are joking).
In contrast to SRT, in the general theory of relativity, mathematical preferences have prevailed over physical meaning, although the consequences of such preferences are not so obvious (mathematicians joke more carefully than physicists).
Currently, the most recognized definition of the essence of general relativity is the expression of the interval:
This expression is interpreted as a change in the properties (measures of length) of space in the presence of masses while maintaining the magnitude of the speed of light.
But if you carefully consider the equation for the interval, realizing that it is not Lorentz invariant, but is valid for any laboratory coordinate system, you can find two ways to explain it - mathematical and physical. The first is based on a geometric method for solving physical problems and is fully implemented in the apparatus of the general theory of relativity and field theories. But the second method, based on the possibility of changing the speed of light in the presence of masses, for some unknown reason, is completely excluded from consideration in physical theories. However, it is the second method that has a clear physical justification, since the phenomenon of light refraction is widely known in optics, caused by a decrease in the speed of propagation of electromagnetic waves in a physical medium; and the presence of a term in this expression can be interpreted both as the presence of a scale factor in nature and as the presence of a refractive index in a vacuum, the value of which in the presence of gravitational masses is different from the value of this parameter in the absence of the indicated masses.
In order to make the right choice, which of the interpretations is satisfactory, we need to figure out what is the cause of the curvature of space - a physical phenomenon or the result of a mathematical description of gravitational interaction.
To do this, it is necessary, first of all, to understand what kind of space we are talking about - a mathematical (mental entity), or a physical (real entity) gravitational field. The fact that physical and geometric quantities are combined in the Einstein's field equation does not yet indicate the physical nature of the curvature of space, since the physical quantities of this equation do not relate to space itself, but to the sources of the gravitational field included in it. And correct, from the standpoint of maintaining the continuity of the coordinate system, on which the formulation of the terms from the left side of the Einstein field equation is based, is the condition for the absence of size at the field sources - a point model of elementary particles. Note that this condition is mandatory for any physical field with its mathematical description by the currently known methods of geometric construction of coordinate space. If the field source has dimensions, then the origin of the associated coordinate system turns out to be inside different from the actual field of the physical entity - another space. In this case, the problem arises exceptions from considering the inner space and replacing it with the outer one. In general relativity, this problem manifests itself when a parameter appears in the solutions of the field equation that quantitatively coincides with the radius holes in the field filled with the substance of the source of this field.
In order to somehow ensure the correspondence of the mathematical model (gravitational field) to physical reality, provided that the continuity of the coordinate system is preserved, it is possible, through the concept of affine connection, to introduce the concept of "curvature" of space in the presence of gravitational masses as a way of mapping space with "holes" on continuous space. But in this case, the curved space is no longer a physical entity, but is a kind of adequate mathematical model.
Thus, the effect of space curvature arises already at the stage of the mathematical description of the gravitational interaction and, in principle, does not require additional physical substantiation.
At the same time, without changing the concepts of space as a linear, homogeneous and continuous entity, which are very convenient for mathematics and everyday thinking, one can use the presence of elementary particles of finite sizes to determine the indicator of the change in the speed of light in the vicinity of gravitational mass as follows:
Since the designations are obvious, it is only necessary to clarify that the radius of a particle with a mass equal to the mass of a proton is taken as the calculated size of an elementary particle, only for convenience in analysis. Of course, this radius will depend on the magnitude of the gravitational field, and we use some average size, which still needs to be determined, preferably based on experimental data. This condition is most consistent with the data on the displacement of the perihelion of Mercury, on the basis of which it is possible to calculate the displacement of the perihelion of other planets and compare them with experimental data. For comparability with the results obtained by the methods of general theory of relativity, and also due to the complexity of finding a direct analytical solution, we will determine the dependence of the refractive index on the distance between the Sun and the planet through the focal parameter, that is, through the arithmetic mean of the reciprocal of the radius at the points of apogee and perigee:
In this case, the magnitude of the perihelion displacement is determined by the expression:
The required average size of the conditional proton will be equal to:
Then for the Earth:
For Venus:
For Icarus:
The amount of light deflected by the Sun is determined as a result of the following:
Then, taking into account the difference in the refractive indices of light on the surface of the Sun and on the Earth's orbit, we have;
It is obvious that the results obtained are in almost complete agreement with the experimental data and the results predicted by the general theory of relativity. Moreover, the data on the deflection of light by the Sun are much more consistent with experiment than the predictions of general relativity.
The advantage of the mathematical model over the physical model of the general theory of relativity is the need to know only two experimental parameters - body mass and distance, while the physical model also requires the value of the radius of the conditional proton. However, if you combine these models, then to define the latter, you can write the expression:
theory of relativity model mathematical physical
The value of the radius of the conditional proton obtained using this formula will differ by only three percent from the value based on experimental data on the magnitude of the deflection of light, but this discrepancy is not too fundamental, since both models (physical and mathematical) are conditional.
Thus, the mathematical model of the gravitational field, based on the principle of curvature of the locus of points, and the physical model, based on the change in the optical properties of vacuum, give approximately the same results. But the validity of the first of these models, predicting the presence of properties in space, determined by the global scale factor, could be proved only if the so-called Г-shaped forms were discovered. However, as recent studies show (see, for example, Astrophysical Journal, 591: 599-622, 2003, July 10), there are no objects in nature that could indicate precisely the curvature of space.
In conclusion, it should be noted that when solving physical problems, it is important to observe the axioms and rules of two disciplines at once - physics and mathematics. Otherwise, small inaccuracies lead to big problems in philosophy.
Bibliography
1. Abers E., Lee B. W., Gauge Theories, Phys. Rep., 9C, 1 (1973)
2. Aharonov Y., Casher A. , Susskind L., Phys. Rev., D5, 988 (1972)
3. Aitchison I.J.R., Relativistic Quantum Mechanics, Macmillan, London, 1972.
4. Altarelli G., Partons in Quantum Mechanics, Phys Rep., 81C, 1 (1982)
5. Arnison G. et al., Intermediate vector boson properties at the CERN super proton synchrotron collider, Geneva, CERN, 1985
6. Bernstein J., Spontaneous Symmetry Breaking, Gauge Theories and All That, Rev. Mod. Phys., 46, 7 (1974)
7. Bilenky S.M., Hosek J., Glashow-Weinberg-Salam Theory of Electro-Weak Interactions and the Neutral Currents, Phys. Rep., 90C, 73 (1982)
8. Bogush A.A., Fedorov F.I., Universal matrix form of first-order relativistic wave equations and generalized Kronecker symbols, Minsk, 1980
9. Bogush A.A., Fedorov F.I., Finite Lorentz transformations in quantum field theory // Rep. Math. Phys., 1977, Vol. 11, no. 1
10. J.R.Bond et al, The Sunyaev-Zel'dovich Effect in CMB-Calibrated Theories Applied to the Cosmic Background Imager Anisotropy Power at l> 2000, Astroph.Journal, 626: 12-30, 2005 June 10
12. Catrol Sean, University of Chicago, Astrophys. Journ., 01.09.00
13. Close F.E., An Introduction to Quarks and Partons, Academic Press, London, 1979
14. Cook N., Exotic Propulsion, Jane's Defense Weekly, 07.24.02
15. Cook N., Anti-gravity propulsion comes out of the closet, Jane's Defense Weekly, 07.31.02
16. Dokshitzer Y.L., Dyakonov D.I., Trojan S.I., Hard Processes in Quantum Chromodynamics, Phys. Rev., 58C, 269 (1980)
17. Dolgov A.D., Zeldovich Y.B., Cosmology and Elementary Particles, Rev. Mod. Phys., 53, 1 (1981)
18. Ellis J., Grand Unified Theories in Cosmology, Phys. Trans. Roy. Soc., London, A307, 21 (1982)
19. Ellis J., Gaillard M.K., Girardi G., Sorba P., Physics of Intermediate Vector Bosons, Ann. Rev. Nucl. Particle Sci. 32, 443 (1982)
20. Ellis J., Sachrajda C.T., In: Quarks and Leptons, NATO Advanced Study Series, Series B, Physics, Vol. 61, Plenum Press, New York, 1979
21. Faddeev L.D., Popov V.N., Phys. Lett., 1967, Vol. 25B, p. thirty
22. Feynman R.P., The Theory of Fundamental Processes, Benjamin, New York, 1962
23. Feynman R.P., Quantum Electrodynamics, Benjamin, New York, 1962
24. Feynman R.P., The Feynman Lectures on Physics, Addison Wesley, Reading, Mass., 1963
25. Feynman R.P., Photon-Hadron Interactions, Benjamin, New York, 1972
26. Feynman R.P., In: Weak and Electromagnetic Interactions at High Energies, Les Houches Session, 29, North-Holland, Amsterdam, 1977
27. Field R.D., In: Quantum Flavordynamics, Quantum Chromodynamics and Unified Theories, NATO Advanced Study Series, Series B, Physics, Vol. 54, Plenum Press, New York, 1979
28. Fradkin E.S., Tyutin I.V., Renormalizible theory of massive vector particles // Riv. Nuovo Cimento, 1974, Vol. 4, no. 1
29. Fritzch H., Minkowski P., Flavordynamics of Quarks and Leptons, Phys. Rep., 73C, 67 (1981)
30. Georgi H., Glashow S.L., Unity of all elementary-particle forces, Phys. Rev. Lett., 1974, Vol. 32, no. 8
31. Georgi H., Lie Algebras in Particle Physics, Benjamin-Cummings, Reading, Mass., 1982
32. Gilman F.J., Photoproduction and Electroproduction, Phys. Rep., 4C, 95 (1972)
33. Glashow S.L., Partial symmetries of weak interactions, Nucl. Phys., 1961, Vol. 22, no. 3
34. Glashow S.L., Illiopoulos I., Maiani L., Weak interactions with lepton-hadron symmetry, Phys. Rev. Series D, 1970, Vol. 2, No. 7
35. Goldstein H., Classical Mechanics, Addison Wesley, Reading, Mass., 1977
36. GoldstoneI., Field theories with “superconductor” solutions, Nuovo Cimento, 1961, Vol. 19, no. 1
37. Green M.B., Surv. High Energy Physics, 3, 127 (1983)
38. Green M.B., Gross D., eds., Unified String Theories, World Scientific, Singapore, 1986
39. Green M.B., Schwarz J.H., Witten E., Superstring Theory, Vol. 1,2, Cambridge University Press, Cambridge, 1986
40. Greene B., The Elegant Universe. Superstrings, Hidden Dimensions, and the Quest for Ultimate Theory, Vintage Books, A Division of Random House, Inc., New York, 1999
41. Halzen Francis, Martin Alan D., Quarks and Leptons. An Introductory Course in Modern Particle Physics, 1983
42. Higgs P.W., Broken symmetries, massless particles and gauge fields, Phys. Lett., Series B, 1964, Vol. 12, no. 2
43. Kac V., Infinite Dimensional Lie Algebras, Bierkhauser, Boston, 1983
44. Kaku M., Introduction to Superstrings, Springer-Verlag, New York, 1988
45. Kim J.E., Langacker P., Levine M., Williams H.H., A Theoretical and Experimental Review of Neutral Currents, Rev. Mod. Phys., 53, 211 (1981)
46. Kobayashi M., Maskawa T., CP violation in the renormalizible theory of weak interactions, Progr. Theor. Phys., 1973, Vol. 49, no. 2
47. Langacker P., Grand Unified Theories and Proton Decay, Phys. Rep., 72C, 185 (1981)
48. Lautrup B., In: Weak and Electromagnetic Interactions at High Energies, NATO Advanced Study Series, Series B, Physics, Vol. 13a, Plenum Press, New York, 1975
49. Leader E., Predazzi E., Gauge Theories and the New Physics, Cambridge University Press, Cambridge, 1982
50. Llewellyn Smith C.H., In: Phenomenology of Particles at High Energy, Academic Press, New York, 1974
51. Moody R.V.J., Algebra, 10, 211 (1968)
52. Mulvey J.H., The Nature of Matter, Clarendon, Oxford, 1981
53. Nambu Y., Lectures at the Copenhagen Summer Symposium, 1970
54. Okubo S., Tosa Y., Duffin-Kemmer formulation of gauge theories, Phys. Rev., 1979, Vol. D20, no. 2
55. Peccei R.D., Status of the standard model, Hamburg, DESY, 1985
56. Politzer H.D., Quantum Chromodynamics, Phys. Rep., 14C, 129 (1974)
57. Polyakov A.M., Phys. Lett., 103B, 207, 211 (1981)
58. Popov V.N., Quantum vortices in the relativistic Goldstone model, Proc. of XII Winter school of theoretical physics in Karpacz, p. 397 - 403
59. Review of particle properties, Particle data group, Geneva, CERN, 1984, Phys. Lett., 1986, Vol. 170B, p. 1 - 350
60. Reya E., Perturbative Quantum Chromodynamics, Phys. Rep., 69C, 195 (1981)
61. Rose M.E., Elementary Theory of Angular Momentum, Wiley, New York, 1957
62. Salam A., Elementary particles theory, Stockholm, W. Swartholm Almquist and Weascell, 1968
63. Schwarz J.H., ed., Superstrings, Vol. 1,2, World Scientific, Singapore, 1985
64. Söding P., Wolf G., Experimental Evidence of QCD, Ann. Rev. Nucl. Particle Sci. 31, 231 (1981)
65. Steigman G., Cosmology Confronts Particle Physics, Ann. Rev. Nucl. Particle Sci. 29, 313 (1979)
66. Steinberg J., Neutrino Interactions, Proc. of the 1976 CERN School of Physics, CERN Rep. 76-20, CERN, Geneva, 1976
67. T'Hooft G., Renormalization Lagrangians for massive Yang-Mills fields, Nucl. Phys. Ser. B, 1971, Vol. 35, no. 1
68. Vilenkin A., Cosmic strings and domain walls, Phys. Rep., 121, 1985
69. Weinberg S., Gravitation and Cosmology, Principles and Applications of the General Theory of Relativity, Mass., 1971
70. Weinberg S., Recent Progress in the Gauge Theories of the Weak, Electromagnetic and Strong Interactions, Rev. Mod. Phys., 46, 255 (1974)
71. Weinberg S., The First Three Minutes, A. Deutsch and Fontana, London, 1977
72. Wiik B.H., Wolf G., Electron-Positron Interactions, Springer Tracts in Mod. Phys., 86, Springer-Verlag, Berlin, 1979
73. Wilczek F., Quantum Chromodynamics, The Modern Theory of the Strong Interaction, Ann. Rev. Nucl. Particle Sci. 32, 177 (1982)
74. Wu T.T., Jang C.N., Phys. Rev., D12, 3845 (1975)
75. Wybourne B.G., Classical Groups for Physicists, Wiley, New York, 1974
76. A. AND. Akhiezer, NS. L. Dokshitser, V. A. Jose... Gluons // UFN, 1980, vol. 132.
77. V. A. Atsyukovsky... Critical analysis of the foundations of the theory of relativity. 1996.
78. J. Bernstein... Spontaneous symmetry breaking // Coll. Quantum theory of gauge fields. 1977.
79. N.N.Bogolyubov, D.V. Shirkov... Quantized fields. 1980.
80. A.A. Bogush... Introduction to gauge field theory of electroweak interactions. 2003.
81. S. Weinberg... Gravity and Cosmology. 2000.
83. V. G. Veretennikov, V. A. Sinitsyn... Theoretical mechanics and additions to general sections. 1996.
84. E. Wigner... Group theory and its applications to the quantum mechanical theory of atomic spectra. 2000.
85. V. I. Denisov, A. A. Logunov... Does gravitational radiation exist in general relativity? 1980.
86. A. A. Detlaf, B. M. Yavorsky... Physics course. 2000.
87. A.D.Dolgov, Ya.B. Zeldovich... Cosmology and elementary particles. // UFN, 1980, vol. 130.
88. V. I. Eliseev... Introduction to the methods of the theory of functions of a spatial complex variable. 1990.
89. V. A. Ilyin, V. A. Sadovnichy, Bl.H. Sendov. Mathematical Analysis, Textbook in 2 parts, 2004
90. E. Cartan... Geometry of Lie groups and symmetric spaces. 1949.
91. F. Close... Quarks and Partons: An Introduction to Theory. 1982.
92. N.P. Konopleva, V.N.Popov... Calibration fields. 2000.
93. A. Likhnerovich... Connection theory in general and holonomy groups. 1960.
94. V. I. Morenko. General theory of relativity and wave-particle dualism of matter. M., 2004.
95. A.Z. Petrov... New methods in general relativity. 1966.
96. A.M. Polyakov... Gauge fields and strings. 1994.
97. Yu.B. Rumer... Research on 5-optics. 1956.
98. V. A. Rubakov... Classic gauge fields. 1999.
99. V. A. Sadovnichy... Operator theory. 2001.
100. A.D. Sukhanov... Fundamental course in physics. The quantum physics. 1999.
101. J. Wheeler... Gravity, neutrinos and the universe. 1962.
102. L. D. Faddeev... Hamiltonian form of the theory of gravitation // Abstracts of the 5th International conference on gravity and the theory of relativity. 1968.
103. R. Feynman... The theory of fundamental processes. 1978.
104. V.A. Fock... Application of Lobachevsky's ideas in physics. 1950.
105. F. Helsen, A. Martin... Quarks and leptons. 2000.
106. A.K. Shevelev... The structure of nuclei, elementary particles, vacuum. 2003.
107. E. Schrödinger... Space-time structure of the Universe. 2000.
108. I.M. Yaglom. Complex numbers and their applications in geometry. 2004.
Home> Document"Paradoxes"
general relativity
As in the special theory of relativity, in general relativity, "paradoxes" allow not only to reject reasoning based on the so-called "common sense" (everyday, everyday experience), but also to give a correct, scientific explanation of the "paradox", which, usually is a manifestation of a deeper understanding of nature. And this new understanding is given by a new theory, in particular, general relativity.
"The twins paradox"When studying SRT, it is noted that the "paradox of twins" cannot be explained within the framework of this theory. Let us recall the essence of this "paradox". One of the twin brothers flies away in a spaceship and, having completed a journey, returns to Earth. Depending on the magnitude of the accelerations that the astronaut will experience during the start, turn and landing, his clock can significantly lag behind the earth's clock. It is also possible that he will not find on Earth either his brother or the generation that he left on Earth at the start of the flight, since more than a dozen (hundreds) years will pass on Earth. This paradox cannot be resolved within the framework of the SRT, since the CRs under consideration are not equal (as is required in the SRT): the spacecraft cannot be considered by the IFR, since it moves unevenly in certain sections of the trajectory.
Only within the framework of general relativity, we can understand and explain the "paradox of twins" in a natural way, based on the provisions of general relativity. This problem is associated with the slowing down of the pace of the clock in moving
CO (or in an equivalent gravitational field).
Let two observers - "twin" are initially on the Earth, which we will consider inertial CO. Let the observer "A" remain on the Earth, and the second observer - "twin" "B" starts on the spaceship, flies away into the unknown expanses of the Cosmos, turns his ship around and returns to the Earth. If the movement in Space is even, then during takeoff, turn and landing, the twin "B" experiences overloads, as it moves with acceleration. These non-uniform movements of the cosmonaut "B" can be likened to his state in some equivalent gravitational field. But under these conditions (in IFR without a gravitational field or in an equivalent gravitational field), a physical (and not kinematic, as in SRT) slowdown of the clock rate occurs. In general relativity, a formula was obtained, which received a specific expression through the gravitational potential:
from which it is clearly seen that the rate of the clock slows down in a gravitational field with a potential (the same is true for an equivalent accelerating CO, which in our problem is a spaceship with a "twin" "B").
Thus, the clocks on Earth will show a longer period of time than the clocks on the spacecraft when it returns to Earth. Another variant of the problem can be considered, considering the "twin" "B" to be motionless, then the "twin" "A" together with the Earth will move away and approach the "twin" "B". An analytical calculation in this case also leads to the result obtained above, although it would seem that it should not have happened. But the fact is that to keep the "spaceship" at rest, it is necessary to introduce holding fields, the presence of which will cause the expected result represented by formula (1).
Let us repeat once again that the "paradox of twins" has no explanation in the special theory of relativity, in which only equal inertial FRs are used. According to SRT, the "twin" "B" must always move away from the observer "A" uniformly and rectilinearly. Popular literature often bypasses the "acute" moment in the explanation of paradox, replacing the physically lasting turn of the spacecraft "back to Earth" by its instantaneous turn, which is impossible. But this "deceptive maneuver" in the reasoning eliminates the accelerated movement of the ship on the turn, and then both COs ("Earth" and "Ship") turn out to be equal and inertial, in which the provisions of the SRT can be applied. But such a technique cannot be considered scientific.
In conclusion, it should be noted that the "paradox of twins" is essentially a variation of the effect called a change in the frequency of radiation in a gravitational field (the period of the oscillatory process is inversely proportional to the frequency, if the period changes, the frequency also changes)
Deflection of light rays passing near the sun
Thus, the results of our expedition leave little doubt that the rays of light are deflected near the Sun and that the deflection, if attributed to the action of the Sun's gravitational field, corresponds in magnitude to the requirements of Einstein's general theory of relativity.
F. Dyson, A. Eddington, C. Devidson 1920
Above is a quote from the report of scientists who observed a total solar eclipse on May 9, 1919 in order to detect the effect of deflection of light rays predicted by general relativity when they pass near gravitating bodies. But let's touch a little on the history of this issue. As you know, thanks to the indisputable authority of the great Newton, in the 18th century. His doctrine of the nature of light triumphed: unlike his contemporary and no less famous Dutch physicist Huygens, who considered light as a wave process, Newton proceeded from the corpuscular model, according to which the particles of light are like material (material) particles interact with the environment in which bodies move and are attracted by the laws of gravity, built by Newton himself. Therefore, light corpuscles should deviate from their rectilinear motion near gravitating bodies.
Newton's problem was theoretically solved in 1801 by the German scientist Zeldner. A quantitative calculation predicted the angle of deflection of light rays when passing near the Sun by a magnitude of 0.87 ".
In general relativity, a similar effect is predicted, but its nature is assumed to be different. Already with SRT, light particles - photons - are without mass particles, therefore the Newtonian explanation in this case is completely unsuitable. Einstein approached this problem from the general idea that a gravitating body changes the geometry of the surrounding space, making it non-Euclidean. In curved space-time, free movement (which is the movement of light) occurs along geodesic lines, which will not be straight in the Euclidean sense, but will be the shortest lines in curved space-time. Theoretical calculations gave a result two times greater than that obtained according to the Newtonian hypothesis. So the experimental observation of the deflection of light rays near the surface of the Sun could also solve the question of the physical reliability of the entire general relativity.
It is possible to check the effect of general relativity by the deflection of light rays by a gravitational field only when light from a star passes near the surface of the Sun, where this field is large enough to significantly affect the geometry of space-time. But under normal conditions, it is impossible to observe a star near the disk of the Sun due to the brighter light from the Sun. That is why scientists used the phenomenon of total solar eclipse, when the disk of the Sun is covered by the disk of the Moon. Einshtein suggested photographing the space around the sun during the minutes of a total solar eclipse. Then the same section of the sky should be photographed again when the Sun is far from it. Comparing both photographs will reveal the shift in the position of the stars. Einstein's theory gives the following expression for the value of this angle:
, (2)
where M is the mass of the Sun. R- the radius of the Sun, G-gravitational constant, WITH- the speed of light.
Already the first observations of this effect (1919) gave a completely satisfactory result: with an error of 20%, the angle turned out to be 1.75 ". In spite of the fact that eclipses happen several times a year, but not always where there are conditions for observation, and the weather (clouds) did not always favor scientists. which distorted the image of the star. And yet it was possible to increase the accuracy and reduce the error to 10%. The situation changed significantly when radio interferometers were created, thanks to the use of which the observation error decreased to 0.01 "(i.e. 0.5 % of 1.75 ").
In the 70s. The deviation of radio beams from quasars (stellar formations, the nature of which has not been sufficiently studied) ZC273 and ZC279 was measured.
The measurements gave the values 1 ", 82 ± 0", 26 and 1 ", 77 ± 0", 20, which is in good agreement with the predictions of general relativity.
So, the observation of the deviation of light (electromagnetic) waves from straightness (in the sense of Euclidean geometry) when passing near massive celestial bodies unambiguously testifies in favor of the physical reliability of general relativity.
Rotation of the perihelion of Mercury
A. Einstein, developing general relativity, predicted three effects, the explanation of which and their quantitative estimates did not coincide with what could be obtained on the basis of the Newtonian theory of gravitation. Two of these effects (the redshift of spectral lines emitted by massive stars and the deflection of light rays when passing near the surface of the Sun and other celestial bodies) were considered above. Consider the third gravitational effect predicted by Einstein - the rotation of the perihelion of the planets of the solar system. On the basis of Tycho Brahe's observations and Kepler's laws, Newton established that the planets revolve around the Sun in elliptical orbits. Einstein's theory made it possible to detect a more subtle effect - the rotation of the ellipses of the orbits in their plane.
Without going into rigorous mathematical calculations, we will show how you can estimate the expected values of orbital rotations. For this, we will apply the so-called method of dimensions. In this method, on the basis of theoretical considerations or experimental data, the values that determine the process under consideration are established. From these quantities, an algebraic expression is compiled, which has the dimension of the desired quantity, to which the latter is equated. In our problem, we will choose as defining quantities:
1) The so-called gravitational radius of the Sun, which for the Sun (and other celestial bodies) is calculated by the formula
2) The average distance of the planet to the Sun
(for Mercury it is equal to 0.58 )
3) Average angular velocity of rotation of the planet around the Sun
Using the method of dimensions, we will compose the following value (it should be noted that the method of dimensions requires the intuition of the researcher, a good understanding of physics, which, as a rule, is given by repeated training and solving similar problems):
where determines the angular velocity of movement of the perihelion of the planet's orbit.
For Mercury (for Earth
). To represent the magnitude of the rotation angle of the planet's perihelion, recall that the angular second is the angle at which the penny coin is "visible" from a distance of 2 km!
The movement of the perihelion of the planet Mercury was first observed long before the creation of general relativity by the French astronomer Le Verrier (19th century), but only Einstein's theory provided a consistent explanation for this effect. It is interesting that scientists managed to "reproduce" this celestial phenomenon by observing the movement of artificial satellites of the Earth. Since the angle of rotation of the perihelion is proportional to the semi-major axis of the satellite's orbit, its eccentricity and is inversely proportional to the orbital period of the satellite, then, choosing the appropriate values of these quantities, we can make = 1500 "in 100 years, and this is more than 30 times the angle orbital rotation for Mercury.However, the task becomes much more complicated, since the movement of an artificial satellite is influenced by air resistance, not the spherical shape and inhomogeneity of the Earth, attraction to the Moon, etc. Yet observation over thousands of artificial satellites, launched into near-Earth space over the past more than 30 years, unambiguously confirm the predictions of general relativity.
Calculating the "radius" of the universe
Among the various models of the Universe considered in general relativity, there is the so-called model of the stationary Universe, first considered by A. Einstein himself. The world turns out to be finite (but unlimited!), It can be represented as a ball (the surface of the ball has no border!). Then it becomes possible to determine the "radius" of such a universe. To do this, we assume that the total energy of the spherical universe is due exclusively to the gravitational interaction of particles, atoms, stars, galaxies, star formations. According to SRT, the total energy of a motionless body is , where M- the mass of the Universe, which can be associated with its "radius" as follows
,
-average density of matter, evenly distributed in the volume of the World. Gravitational energy of a spherical body of radius
can be calculated elementarily and is equal to:
Neglecting the numerical coefficients of the order of unity, equating both expressions for energy, we obtain the following expression for the "radius" of the Universe:
Taking (which is consistent with observations)
we get the following value for the "radius" of the World:
This value determines the visible "horizon" of the World. Outside this sphere, there is no matter and electromagnetic field. But immediately new problems arise: what about space and time, do they exist outside the sphere? All these questions have not been resolved, science does not know an unambiguous answer to such questions.
The "finiteness" of the Universe in the considered model removes the so-called "photometric paradox": the night sky cannot be bright (as it should be if the Universe is infinite and the number of stars is also infinite), since the number of stars (according to the considered model ), of course, due to the finiteness of the volume of the World, and due to the absorption of the energy of electromagnetic waves in interstellar space, the illumination of the sky becomes small.
The model of the stationary Universe is the very first model of the World, as mentioned above, proposed by the creator of general relativity. However, already at the beginning of the 20s. Soviet physicist and mathematician A.A. Fridman gave another solution to Einstein's equations in general relativity and received two variants of development for the so-called nonstationary universe. A few years later, the American scientist Hubble confirmed Friedman's decisions by discovering the expansion of the Universe. According to Friedman, depending on the value of the average density of matter in the Universe, the currently observed expansion will either continue forever, or after the slowdown and stop of galactic formations, the process of contraction of the World will begin. Within the framework of this book, we cannot further discuss this topic and refer curious readers to additional literature. We touched on this issue because the model of the expanding Universe also allows us to eliminate the photometric paradox discussed above, relying on other grounds. Due to the expansion of the Universe and the distance of stars from the Earth, the Doppler effect should be observed (in this case, a decrease in the frequency of the incoming light) - the so-called redshift of the frequency of light (not to be confused with a similar effect associated not with the movement of the light source, but with its gravitational field). As a result of the Doppler effect, the energy of the light flux is significantly weakened and the contribution of stars located outside a certain distance from the Earth is practically zero. At the present time, it is generally accepted that the Universe cannot be stationary, but we used such a model due to its "simplicity", and the obtained "radius" of the World does not contradict modern observations.
"Black holes"
Let's say right away that "black holes" in the Universe have not yet been experimentally discovered, although there are up to several dozen "candidates" for this name. This is due to the fact that a star that has turned into a "black hole" cannot be detected by its radiation (hence the name "black hole"), since, having a giant gravitational field, it does not give either elementary particles or electromagnetic waves leave their surface. Many theoretical studies have been written devoted to "black holes", their physics can be explained only on the basis of general relativity. Such objects can appear at the final stage of a star's evolution, when (at a certain mass, not less than 2-3 solar masses) the light radiation pressure cannot counteract the gravitational compression and the star experiences a "collapse", i.e. turns into an exotic object - a "black hole". Let's calculate the minimum radius of the star, starting from which its "collapse" is possible. For a material body to be able to leave the surface of a star, it must overcome its attraction. This is possible if the body's own energy (rest energy) exceeds the potential energy of gravity, which is required by the law of conservation of total energy. You can make up the inequality:
Based on the principle of equivalence, the left and right are the same body weight. Therefore, up to a constant factor, we get the radius of a star that can turn into a "black hole":
For the first time this value was calculated by the German physicist Schwarzschild back in 1916, in honor of him this value is called the Schwarzschild radius, or gravitational radius. The sun could turn into a "black hole" for the same mass, with a radius of only 3 km; for a celestial body equal in mass to the Earth, this radius is only 0.44 cm.
Since the formula for , the speed of light enters, then this celestial object has a purely relativistic nature. In particular, since the physical deceleration of the clock in a strong gravitational field is affirmed in general relativity, this effect should be especially noticeable near the "black hole". So, for an observer outside the gravitational field of a "black hole", a stone falling freely on a "black hole" will reach the Schwarzschild sphere in an infinitely long period of time. While the watch of the "observer", falling along with the stone, shows the end (proper) time. Calculations based on the positions of general relativity lead to the fact that the gravitational field of the "black hole" is not only capable of curving the trajectory of a light beam, but also to capture the light flux and make it move around the "black hole" (this is possible if the light beam passes through at a distance of about 1.5, but this movement is unstable).
If the collapsed star had angular momentum, i.e. rotated, then the "black hole" must retain this rotational moment. But then around this star and the gravitational field should have a vortex character, which will manifest itself in the originality of the properties of space-time. This effect could make it possible to detect a "black hole".
In recent years, the possibility of "evaporation" of "black holes" has been discussed. This is due to the interaction of the gravitational field of such a star with the physical vacuum. In this process, quantum effects should already be felt, i.e. General relativity turns out to be connected with the physics of the microworld. As you can see, the exotic object predicted by general relativity - the "black hole" - turns out to be a connecting link of seemingly distant objects - the microworld and the Universe.
Additional reading literature
1. Braginsky V.B., Polnarev A.G. Amazing gravity M., Mir, 1972.
In fact, we have already begun to analyze the paradoxes of SRT. The structure of the linear paradoxes of STR is standard, and it can be illustrated by the following example.
Have two gentlemen of the same height enter different rooms separated by a transparent partition. They don't know that the septum is a biconcave lens. The first gentleman claims to be taller than his colleague. The second, comparing his height with the apparent height of a colleague, asserts something opposite. Which one is right? Which one is actually higher?
Now the answer is obvious to us. It is wrong to compare the characteristic of the essence (own growth) with the characteristic of the phenomenon (observed, apparent growth), interpreting it as "essence". The characteristics of an entity can be distorted when displayed in the observer's frame of reference.
Rice. 2.
Let's move on to the paradoxes of SRT, using the “golden rule”. Recall that the condition in SRT is the speed of relative motion. Everything that depends on this speed is a characteristic of the phenomenon.
Slowing down time. Let's go back to the rather annoying twins paradox. The stationary brother sees that the pace of life of the moving brother is slower. In his frame of reference, a moving brother observes a similar phenomenon: it seems to him that the pace of his brother's life is slower and that he is "younger". The "slowdown" of the tempo depends on the magnitude of the speed of the relative movement. It is a phenomenon. Due to the equality of reference systems, the phenomena observed by each of the brothers are the same (symmetric) and we get the logical contradiction of SRT (SRT paradox).
This paradox is easily resolved if we divide the effects into phenomenon and essence. In this situation, we must admit, firstly, that the phenomena are really the same (symmetric). Secondly, the actual tempo of time does not depend on the choice of the frame of reference by the observer (any of the brothers), i.e. time is the same for all frames of reference. The observed "slowing down" of the rate of time is the usual Doppler effect. And no problem! Everything is exactly the same as in the case of gentlemen.
Compression of scale. The structure of the paradox is standard. Let the twins stand perpendicular to the relative velocity vector. Then each of the twins will see his brother thin ("refined")! But if they get tired and lie along the vector of this speed, they will find that the observed moving brother will look "shortened". The observed "shortening" is due to the distortion of the front of the light wave when the light beam passes from one frame of reference to another. The essence of the paradox is the same, and there is no need to "braid" another theory (GTR) to explain it. It is necessary to correctly apply the theory of knowledge to physics.
Have you ever seen how fun the kids from kindergarten laugh when they visit the “laugh room” with crooked mirrors? They know nothing about "phenomena and entities." But they are well aware that the distorted figures they observe are "hocus-pocus" (pretend). They know very well that they do not "crooked", but remain the same as they were, in contrast to the dogmatic "academicians-relativists".
Lenin and Mach. Now we will show the "stump" that Ernst Mach, the idol of A. Einstein, stumbled over. IN AND. Lenin in his book "Materialism and Empirio-Criticism" harshly criticizes his philosophical conclusions. We would like to draw your attention to the starting point that marked the beginning of Mach's error. We quote Lenin's Materialism and Empirio-Criticism:
“We saw that Marx in 1845, Engels in 1888 and 1892. introduce the criterion of practice into the basis of the theory of knowledge of materialism. Outside of practice, it is scholasticism to pose the question of whether “objective” (ie, objective) “truth” corresponds to human thinking, ”says Marx in the second thesis on Feuerbach. The best refutation of Kantian and Humean agnosticism, as well as other philosophical quirks (Schrullen), is practice, ”Engels repeats. "The success of our actions proves the agreement (correspondence, bereinstimmung) of our perceptions with the objective (objective) nature of perceived things," Engels objects to agnostics.
Compare this with Mach's reasoning on the criterion of practice. “In everyday thinking and everyday speech, the usually seeming, illusory reality is opposed. Holding a pencil in front of us in the air, we see it in a straight position; lowering it in an inclined position into the water, we see it bent. In the latter case, they say: "the pencil appears to be bent, but in reality it is straight." But on what basis do we call one fact reality, and reduce the other to the value of an illusion? .. When we make the natural mistake that in extraordinary cases we still wait for the onset of ordinary phenomena, our expectations, of course, are deceived. But the facts are not to blame for this. Talking about illusion in such cases makes sense from a practical point of view, but not at all scientific. To the same extent, the frequently discussed question, whether the world really exists, or is it just our illusion, no more than a dream, does not make any sense from the scientific point of view. But even the most incongruous dream is a fact, no worse than any other ”(“ Analysis of Sensations ”, pp. 18 ... 19).
Now the floor is to us. We are considering a "pencil", and the pencil we see is a phenomenon. Looking from the end, we will see a hexagon, and looking from the side, we will see a rectangle. If we dip the end of the pencil obliquely into a glass of water, we will see it “broken”. All these are phenomena behind which the essence was hidden from Mach. Mach got confused, not knowing the criteria for distinguishing phenomenon from essence, and, as a result, fell into idealism.
Lenin writes there:
"This is precisely such a tortured professorial idealism, when the criterion of practice, which separates the illusion from reality for each and every one, is carried out by E. Mach outside the bounds of science, outside the bounds of the theory of knowledge."
To separate the illusion from reality means to separate the phenomenon and the essence, i.e. show: where is the phenomenon, and where we are talking about the essence.
So, we return to the position of classical theories. In them, time for all inertial systems is the same, space is common, and inertial systems are equal!
Unfortunately, stubborn relativists are not convinced by the conclusions of the theory of knowledge of scientific truth (philosophical ignorance!). They will immediately recall the Lorentz transformation, Einstein's thought experiments, point out that within the framework of SRT, time depends on the choice of the frame of reference, they will “broadcast” about the “complete confirmation” of SRT by experiments, etc. Don't worry, gentlemen: “You will have a squirrel and a whistle! ... Pleshcheev A.N. Poem "The Old Man", 1877 "
- 1. As we have established, SRT paradoxes (time dilation, scale contraction, etc.) are common logical contradictions.
- 2. Logical contradictions in the explanation of the Lorentz transformation are due to unfamiliarity with the materialistic theory of cognition of scientific truth and, in particular, with the wrong classification and correlation of physical phenomena with the philosophical categories of "phenomenon and essence". This "suffered" A. Einstein and his idol E. Mach.
- 3. Ignorance and misinterpretation of the content of the categories "phenomenon and essence" is characteristic not only of the beginning of the 20th century. Rarely does any of the modern physicists and philosophers “sin” with knowledge and possession of the methods and criteria of the theory of knowledge (“holy emptiness”).
- 4. Epistemological analysis showed the possibility of giving a new explanation of the essence of the Lorentz transformation within the framework of classical ideas about space and time. Space is common to all inertial reference frames without exception, and time is the same for these inertial frames.
- 5. Below we will continue the analysis and search for a new explanation of the essence of the Lorentz transformation.
The main "purpose" of the set of paradoxes of SRT is to show the internal contradictions of the theory. If a theory makes predictions about a phenomenon that contradict each other, then this indicates that the theory is erroneous, which requires its revision. The paradoxes of SRT are derived from thought experiments, that is, an imaginary experiment based on the provisions of the theory. One of these paradoxes is rightfully considered one of the oldest paradoxes - the Ehrenfest paradox from 1909, nowadays often formulated as the "wheel paradox" and which, according to many authors, has not yet had a satisfactory explanation or solution.
There are several different formulations of Ehrenfest's "paradox" in the literature. Here the word paradox is put in quotation marks deliberately, since in this note it will be shown that the paradox was formulated with errors, based on statements attributed to the special theory of relativity, but which it does not. In general, these different formulations of the paradox can be reduced to three groups:
- when the wheel rotates, the spokes are deformed;
- it is impossible to spin a wheel made of absolutely solid material at all;
- when spinning at light speed (rim), the wheel contracts to a point, disappears.
All these formulations are inherently close enough to each other and, under certain conditions, are combined. For example, in the work "Theory of relativity in an elementary presentation" the following formulation is given:
At first, the wheel is motionless, and then it is set in such a fast rotation that the linear speed of its edges approaches the speed of light. In this case, the sections of the rim ... are shortened .., while the radial "spokes" ... retain their length (after all, only longitudinal dimensions, ie dimensions in the direction of motion, experience relativistic shortening).
Rice. 1. Illustration for the wheel paradox at work
And then the solution to the formulated paradox is given:
When the wheel, which was initially stationary, is set in rapid rotation: its rim tends to contract, and the spokes tend to maintain a constant length. Which of these trends will prevail depends entirely on the mechanical properties of the rim and spokes; but there will be no rim shortening without a proportional shortening of the spokes (unless the wheel takes the form of a spherical segment). Obviously, from a fundamental point of view, nothing will also change if the spoked wheel is replaced by a solid disc. "
The essence of the solution, as we can see, is that either the spokes will necessarily be reduced, or the rim will be extended, depending on the stiffness of the material. Apparently, with the homogeneity of the material, the reduction will be mutual: both the spokes and the rim will contract, but to a lesser extent.
The wheel paradox in Ehrenfest's version is given in the work "Uncorrected Poincaré's error and analysis of SRT":
Consider a flat, solid disc rotating around its axis. Let the linear speed of its edge be comparable in order of magnitude with the speed of light. According to the special theory of relativity, the length of the edge of this disk should undergo a Lorentz contraction ...
There is no Lorentz contraction in the radial direction; therefore, the radius of the disk must retain its length. With such a deformation, the disc technically cannot be flat any more.
The angular velocity of rotation decreases with increasing distance from the axis of rotation. Therefore, the adjacent layers of the disk should slide relative to each other, and the disk itself will experience torsional deformation. The disc should collapse over time.
The interpretation, it should be noted, is very specific: the destruction is associated not with the compression of the inner layers or spokes, but with their bending, twisting. The author does not explain the reason for the occurrence of the difference in angular velocities, referring to Ehrenfest, and only adding:
The relativists themselves could not provide any explanations for the physical reasons, either to explain the hypothesis, or to explain the paradox.
However, this is the only description of the spinning effect that I have come across on the Internet during a cursory scan.
Wikipedia describes the paradox as follows, citing a link to a children's encyclopedia in the text:
Consider a circle (or hollow cylinder) rotating around its axis. Since the speed of each element of the circle is directed tangentially, then it (the circle) must undergo a Lorentz contraction, that is, its size for an external observer must seem less than its own length.
The initially motionless rigid circle, after its untwisting, must paradoxically decrease its radius in order to maintain its length.
According to Ehrenfest's reasoning, an absolutely rigid body cannot be brought into rotational motion, since there should be no Lorentz compression in the radial direction. Consequently, the disk, which was flat at rest, must somehow change its shape during unwinding.
Here, another manifestation of the paradox is indicated with reference to Ehrenfest: an absolutely hard disk cannot be brought into rotation at all. A similar interpretation is given in the "Encyclopedia for Children", which, in turn, refers to the author's work of Ehrenfest - a short note "Uniform rotational motion of bodies and the theory of relativity" from 1909:
The note contained a paradoxical statement: it is impossible to bring an absolutely rigid cylinder (or disk) into rapid rotational motion around the central axis, otherwise a contradiction of the special theory of relativity arises. Indeed, let such a disk rotate, then the length of its circumference will decrease due to Lorentz contraction, and the radius of the disk will remain constant ... In this case, the ratio of the circumference of the disk to its diameter is no longer equal to the number n. This thought experiment is the content of the Ehrenfest paradox.
Here, we can say, is the main, generally accepted formulation of the Ehrenfest paradox, which differs from the common formulation of the wheel paradox. It no longer talks about the deformation of the disc or wheel spokes. The disc will simply remain stationary.
Let's experiment with a disc. We will rotate it, gradually increasing its speed. The size of the disk ... will decrease; in addition, the disc will bend. When the speed of rotation reaches the speed of light, it will simply disappear. And where will he go? ...
The disk should have been deformed during rotation, as shown in the figure.
That is, as above, the conclusion is made about the deformation of the spokes, while, obviously, it is quite reasonably assumed that the hardness of the rim exceeds the flexibility of the spokes.
Finally, in order to find out which of the formulations of the paradox corresponds to the author's, we give a description of the paradox, as it is formulated in the aforementioned work of Ehrenfest. The quote below is practically the entire content of that short note:
Both definitions of non-absolute hardness are - if I understood correctly - equivalent. Therefore, it is enough to point to the simplest form of motion, for which this initial definition already leads to a contradiction, namely, uniform rotation around a fixed axis.
Indeed, let there be a not absolutely rigid cylinder C with radius R and height H. Let it gradually rotate around its axis, which then occurs at a constant speed. Let's call R "the radius that characterizes this cylinder from the point of view of a stationary observer. Then the value of R" must satisfy two conflicting requirements:
a) the circumference of the rotating cylinder in comparison with the state of rest should be reduced:
2πR ′< 2πR,
since each element of such a circle moves in the tangent direction with instantaneous speed R "ω;
b) the instantaneous speed of any element of the radius is perpendicular to its direction; this means that the elements of the radius do not undergo any contraction compared to the state of rest.
Hence it follows that
Comment. If we assume that the deformation of each element of radius is determined not only by the instantaneous velocity of the center of gravity, but also by the instantaneous angular velocity of this element, then it is necessary that the function describing the deformation contains, in addition to the speed of light s, one more universal dimensional constant, or it must contain include the acceleration of the element's center of gravity.
As we can see, at least in the original author's version, the paradox directly concerns not absolutely rigid bodies. It says nothing about curling the layers. Nothing about "disappearing" the disc. Perhaps all these extensions of the original idea were formulated somewhere in the subsequent works of Ehrenfest, but let's leave it all on the conscience of the cited authors: they did not provide verified references to their statements. Thus, we can reasonably consider:
The myth of the Ehrenfest paradox
Consider, if possible, the modern versions of the paradox indicated at the beginning of the article. The simplest and, apparently, the most widespread, is the version of the "wheel paradox", with which, as you can see, the contradiction formulated in 1909 by Ehrenfest most closely coincides. In fact, the paradox of Ehrenfest is exactly the paradox of the wheel.
However, first we will look at its ultimate version. This is a version in which the spokes or the inside of the wheel do not rotate at all. In this case, we get rid of any doubts about whether the needles are shortened or not. Such a "wheel", as you might guess, looks like a hollow thin-walled cylinder or a thin ring, mounted on a thick axle. The solution to this "paradox" is obvious. And again, as above, the word "paradox" is here in quotation marks solely because it is, in fact, not a paradox, but a pseudo-, imaginary paradox. The special theory of relativity describes the behavior of such a wheel without any contradictions. Indeed, from the point of view of the stationary axis, the "rim" of the wheel undergoes a Lorentz contraction during rotation, which leads to a decrease in its diameter. From this point of view, either the wheel will burst, or it will compress the axle, squeezing out a notch on it, or with sufficient elasticity, the ring will stretch. In this case, an external observer will not notice any changes, even if the wheel-ring is spun up to light speed: if only the material of the wheel has enough elasticity.
Now let's go to the frame of reference of the wheel-rim. Obviously, it is impossible to tie the rest system to the entire wheel, since the velocity vectors of the points are directed in different directions. At rest, there can be only one point at a time touching a fixed surface. It is clear that such a "stationary" wheel is just a wheel rolling on a stationary surface. About him, we can only say that the speed of its center is equal to half the speed of the element on the top. But this remark suddenly unexpectedly reminds us of the already considered paradox - the paradox of the transporter. Indeed, in that paradox there are also three points: fixed; the upper one, moving at a certain speed and the middle one, moving at half the speed of the upper one. What can be in common between a wheel and a conveyor?
However, let's take a closer look. Let's look at the wheel at an angle to its axis. The larger this angle, the more "flattened" the wheel, taking the form of an elongated ellipse, which quite noticeably resembles a conveyor.
Rice. 2. When viewed from a large angle, the wheel looks like an ellipse. The thickened circle is the outer surface of the wheel axle. Thin line circle - rotating rim (wheel)
Although on the resulting conveyor belt - the wheel rim moves along an elliptical trajectory, we may well consider the "projection" of this rim onto the horizontal axis. In this case, we get a perfectly admissible analogy of the conveyor belt problem and its obvious solution:
In both cases, both from the point of view of the beam (bed) and from the point of view of ... the belt, the result will be a tension on the belt, leading either to deformation of ... of the bed, or to deformation of ... of the belt. Depending on the initial conditions: which will be set more durable. The transporter paradox turned out to be an imaginary, seeming paradox.
The wheel rim, visible as a conveyor belt, as in the conveyor problem, will contract, which will inevitably lead either to its rupture or to deformation of the axle, which looks like a conveyor frame at a selected angle. It is clear that the axle can be segmented, that is, consist of spokes, which, like the solid axle, will be deformed if the rim turns out to be stronger.
Thus, the variant of the "paradox" of a wheel with a thin rim and a fixed axle is not a paradox, since the theory of relativity makes consistent predictions about it.
Now let's move on to the solid disc. Moreover, we will consider it absolutely solid, that is, consider a variant of the Ehrenfest paradox about the impossibility of spinning such a disk.
Imagine a disc as concentric circles piled on top of each other - rims of a fairly small thickness and rigidly fastened to each other. Let us denote the radius of each such rim by Ri. The circumference of each rim is respectively 2πRi. Let's say we managed to spin up the disk. The angular velocity of the disc ω is the same for each point of the disc and determines the linear velocity of each particular rim of the disc. Here we strongly reject the idea of twisting as unfounded. The tangential speed of each point of the rim is vi = ωRi. The shortened circumference of each rim is determined by the Lorentz equations:
L i= 2 π R i1 − ω 2R 2 i−−−−−−−−√ Li = 2πRi1 − ω2Ri2
Here we consider the problem in the system of units, in which the speed of light is c = 1. Consider two rims: the outer one with R0 and one of the inner ones - R1, let R1 = kR0, where k = 0 ... 1. From equation (1) we obtain:
L 1= 2 π k R 01 − ω 2k 2R 2 0−−−−−−−−−√ L 0= 2 π R 01 − ω 2R 2 0−−−−−−−−√ L1 = 2πkR01 − ω2k2R02L0 = 2πR01 − ω2R02
When the disc was “unwound”, these two rims decreased their length. Therefore, the radii of their new circles will be:
lR 1 ω= L 12 π= k R 01 − ω 2k 2R 2 0−−−−−−−−−√ R 0 ω = L 02 π= R 01 − ω 2R 2 0−−−−−−−−√ lR1ω = L12π = kR01 − ω2k2R02R0ω = L02π = R01 − ω2R02
The ratio of the rim radii after spinning is:
R 1 ωR 0 ω = k R 01 − ω 2k 2R 2 0−−−−−−−−−√ R 01 − ω 2R 2 0−−−−−−−−√ = k 1 − ω 2k 2R 2 01 − ω 2R 2 0−−−−−−−−−−√ R1ωR0ω = kR01 − ω2k2R02R01 − ω2R02 = k1 − ω2k2R021 − ω2R02
This expression shows that the ratio of the radii of adjacent layers depends on the rotation speed. We should be interested in what the rotation speed can be so that the radii that differ by a factor of k in a stationary state become equal after the spin. Apparently, this will be the limiting speed, after which the layers will "creep" on top of each other. Let's calculate this ratio for the specified condition:
R 1 ωR 0 ω = k 1 − ω 2k 2R 2 01 − ω 2R 2 0−−−−−−−−−−√ = 1 R1ωR0ω = k1 − ω2k2R021 − ω2R02 = 1
For clarity, let's drop the left equality:
k 1 − ω 2k 2R 2 01 − ω 2R 2 0−−−−−−−−−−√ = 1 k1 − ω2k2R021 − ω2R02 = 1
Divide everything by k
1 − ω 2k 2R 2 01 − ω 2R 2 0−−−−−−−−−−√ = 1 k 1 − ω2k2R021 − ω2R02 = 1k
Square both sides of the equality
1 − ω 2k 2R 2 01 − ω 2R 2 0= 1 k 2 1 − ω2k2R021 − ω2R02 = 1k2
Getting rid of the fractional view
k 2− ω 2k 4R 2 0= 1 − ω 2R 2 0 k2 − ω2k4R02 = 1 − ω2R02
Move the terms with radii to the left, and to the right terms without radii
ω 2R 2 0− k 4ω 2R 2 0= 1 − k 2ω2R02 − k4ω2R02 = 1 − k2
Collecting similar members
ω 2R 2 0(1 − k 4) = 1 − k 2ω2R02 (1 − k4) = 1 − k2
Rewrite the equation as a solution for the radius term
ω 2R 2 0= 1 − k 21 − k 4ω2R02 = 1 − k21 − k4
We see that on the right in the equality there are canceled terms
ω 2R 2 0= 1 − k 2(1 − k 2) (1 + k 2) ω2R02 = 1 − k2 (1 − k2) (1 + k2)
Reducing
ω 2R 2 0= 1 1 + k 2ω2R02 = 11 + k2
Replace angular velocity with linear velocity
v 2 0= 1 1 + k 2 v02 = 11 + k2
Extract the root and find the speed value
v 0= 1 1 + k 2−−−−−√ v0 = 11 + k2
The intersection can begin between adjacent layers, for which almost k = 1. The actual intersection occurs at the speed of the outer rim:
v 0= 1 1 + 1 −−−−√ = 1 2 –√ = 2 –√ 2 ≈ 0 , 7 v0 = 11 + 1 = 12 = 22≈0.7
First, it means that our assumption about the possibility of spinning the disk turned out to be valid. Secondly, we find that two adjacent infinitely thin layers-rims will press on each other only when their speed is more than 0.7 times the speed of light. And this, in turn, means that when unwinding, each rim decreases both the length of its circumference and the corresponding radius. Thus, here we find the misconception regarding the reduction of the spokes of a rotating wheel. When formulating the paradox, all authors explicitly state that the rim contracts, but the spokes do not. We found, on the contrary, that each rim, each thin layer of the wheel shrinks and decreases its own radius. Therefore, it does not interfere with the contraction of the layer, the rim, which is located above it. In the same way, the layer, the rim below it, does not hinder its own compression either. Since the considered rims all together form a solid disk of the wheel, this wheel as a whole does not experience any internal deformations that prevent its compression. The statements of all authors, including the author of the paradox - Ehrenfest - are erroneous: the radius of the wheel will decrease without any obstacles:
Radius elements do not undergo any contraction compared to the resting state.
But the detected contraction, contraction of radii has a rather strange feature: this contraction is possible only up to the tangential speed of the outer rim, which does not exceed 0.7 of the speed of light. Why exactly 0.7? Where, from what physical features of the wheel does this number come from? And what happens if you spin the wheel even faster?
However, why do we argue that the spokes will shorten, because there are no spokes in our model, the wheel is solid. And in a wheel with spokes there are no "thin rims", there is empty space between adjacent spokes.
As correctly stated in the work, there is no difference between a solid wheel and a spoke wheel. All elements distant from the center at the same distance are subject to the Lorentz contraction. That is, in this case, the "thin layer" is a sequence of "lobules" of the spokes and the empty space between them. A bewildered objection may arise here: how is it, why is each "slice" of the spoke being compressed along the circumference? After all, they have empty space next to them! Yes, empty. But all elements, without exception, are subject to Lorentz contraction, this is not a real physical contraction, this is a contraction visible to an external observer. As a rule, when describing the Lorentz contraction, it is always emphasized: from the point of view of an external observer, it has reduced its size, although from the point of view of the object itself, nothing happened to it.
To explain this tangential compression, thinning of the spokes, imagine a moving platform on which, for example, bricks are laid at intervals. To an outside observer, it will appear that the platform has shrunk. And what will happen with the intervals between the bricks? The bricks, of course, will shrink, but if the interval between them remains unchanged, they will simply push each other off the platform. However, in reality, the bricks and the intervals between them are reduced as one single object. Any observer moving past the platform will see its reduced length, depending on the relative speed, and the reduced length of the "spaced bricks" object. As you know, nothing will happen with the platform itself, the bricks and the intervals between them.
So it is with the spoked wheel. Each separate radial layer of the wheel - the rim - will be a "puff pie" consisting of successive pieces of spokes and the space between them. By shortening in length, such a "layered" rim will simultaneously decrease its radius of curvature. In this sense, it is useful to imagine that the wheel is first spun, then slowed down to a stop. What will happen to him? It will return to its original state. The decrease in its size has nothing to do with its physical deformation, it is the size visible to an external, motionless observer. In this case, nothing happens to the wheel itself.
From this, by the way, it immediately follows that the wheel can be absolutely rigid. No deformation forces are applied to it, changing its diameter does not require direct physical compression of the wheel material. You can spin the wheel, then slow it down as many times as you like: for the observer, the wheel will reduce its size and restore them again. But on one condition: the tangential speed of the outer rim of the wheel should not exceed the mysterious value - 0.7 speed of light.
Obviously, when this speed is reached by the outer rim of the wheel, the speeds of all those below it will obviously be lower. Consequently, the "wave" of overlap will start from the outside and will gradually move inside the wheel, towards its axis. Moreover, if the outer rim is spun up to the speed of light, the layers will overlap only up to the layer with 0.7 of the original wheel radius. All layers closer to the axis will not overlap each other. It is clear that this is a hypothetical model, since it is not yet clear what will happen to the layers located further from the axis than 0.7 of the original radius. Let us recall the exact value of this quantity: √2 / 2.
The diagram shows the process of reducing the radii of the layers and the starting point of their intersection:
Rice. 3. Compression ratios of the rim radii depending on their distance from the center and the tangential speed of the outer rim
With an increase in the tangential velocity of the outer edge of the disk, its layers - the rims decrease their own radii to varying degrees. The radius of the outer edge decreases the most, down to zero. We see that the rim, the radius of which is equal to a tenth of the radius of the outer edge of the disk, practically does not change its radius. This means that with a strong spin, the outer rim will shrink to a radius smaller than the inner one, but how this will look in reality is not yet clear. So far, it is only obvious that deformation occurs only at the speed of the outer rim exceeding √2 / 2 the speed of light (approx. 0.71 s). Up to this speed, all the rims are compressed without crossing each other, without deforming the plane of the disk, the outer radius of which will decrease to 0.7 from the initial value. To illustrate this point, the diagram shows two adjacent outer rim layers that have nearly the same radii. These are the first "candidates" for mutual intersection during unwinding.
If uniformly concentric circles are applied to the disk, at equal intervals, then in the process of its unwinding for an external observer, these circles will be located at intervals that decrease uniformly from the center (almost the initial value of the interval) to the periphery (decreasing to zero).
In order to find out what happens to the wheel after the outer rim exceeds the speed of 0.7 times the speed of light, we will change the shape of the wheel so that the layers do not interfere with each other. Let's shift the layers of the wheel along the axis, turning the wheel into a thin-walled cone, a funnel. Now, when compressing each layer, there are no other layers under it, and nothing prevents it from shrinking as much as necessary. Let's start to unwind the cone from a state of rest to a speed of 0.7 times the speed of light and then to the speed of light, after which we decrease the speed in the reverse order. Let's depict this process in the form of animation:
Rice. 4. Lorentzian deformation of the cone during unwinding. On the left is a view along the axis of the cone - funnels, on the right - a side view, perpendicular to the axis. Red thin line on the cone shows its outline
In the figure, the cone (funnel) is shown in two views: along the axis, as always the paradox of the wheel, and perpendicular to the axis, a side view, which shows the "profile" of the cone. In the side view, we can clearly see the behavior of each cone rim layer, the former wheel. Each of these layers is depicted with a colored line. These lines repeat the corresponding circles, rims, for which the graph is plotted in the previous figure. This allows you to see each rim independently of the others and how the outer rim reduces its radius more than the inner rims.
The following obvious circumstances should be especially noted. According to the theory of relativity, there is no deformation of the disk or the shown cone as such. All changes in its shape are visible to an external observer, while nothing happens to the disc itself and the cone. Therefore, it may well be made of an absolutely solid material. Products made from such material do not shrink, stretch, bend or twist - they are not subject to any geometric deformation. Therefore, the appearance of deformation is quite possible for the spinning of this disk to the speed of light. An outside observer will see, as shown in the animation, a completely logical, albeit rather strange picture. The outer rim of the cone decreases to a speed of 0.7 s, after which it continues to contract further. In this case, the inner rim, which had a smaller radius, turns out to be on the outside. However, this is quite an obvious phenomenon. The colored rims in the animation show how the outer rims approach the center of the disc, transforming the cone into a kind of closed vessel, an amphora. But you need to understand that in this case, the actual cone remains the same as it was originally. If you reduce the speed of its rotation, then all the layers will return to their places and the amphora will again turn into a cone for a stationary observer. From the point of view of an external observer, this apparent displacement of layers, rims due to compression towards the center of the disk has nothing to do with the real geometric deformation of the disk itself. That is why there are no physical obstacles for the cone to be made of an absolutely solid material.
But this applies to the cone. And how will the flat wheel behave, in which all the layers are still one above the other? In this case, a stationary observer will see a very strange picture. After the outer rim of the disc has decreased at a speed of 0.7 s, it will attempt to compress further. In this case, the inner rim, which had a smaller radius, will resist this. Here we recall the obvious condition - the disk must remain flat at any speed.
For all the strangeness of the picture, one can quite easily guess what will happen next. You just need to remember the picture discussed above with a thin-walled wheel mounted on a fixed axle. The only difference is that, in the case under consideration, the stationary axis does not undergo Lorentz contraction. Here, the layers, it is zero to 0.7 of the radius of the wheel, have themselves experienced compression and somewhat reduced their size. Despite this, the outer layers still "caught up" with them. Now the Lorentzian compression of the inner layers is not enough, they do not allow the outer ones to continue their own compression. As options, we can distinguish three scenarios for the further development of events, without taking into account the action of centrifugal forces and the fact that such a spin will require an infinitely powerful engine.
For ordinary material, when the layers-rims interact, the inner layers experience compression deformation, and the outer ones - tension. Consequently, the outer rims are more likely to rupture than the inner rims are elastic. This is obvious, since the material is the same.
Rice. 5. Lorentzian deformation of a disc made of an ordinary solid material
Here and on the following animations, the stripes are painted like a "vest" - lighter colors alternate with darker ones. In this case, when the disc is compressed, it is better seen in its section that they do not intersect each other, but rather fold in the form of an "accordion". In the animation of the compression of an ordinary hard (fragile) disk, the layers (rims) are repainted in red, which come into close contact and press against each other with force. In this case, their material experiences both compressive forces (inner layers) and tensile forces (outer layers). With some effort, the outer layers, which are more likely, will simply be torn apart and scatter in different directions. As you can see in the animation, the conditions for rupture occur after reaching the maximum speed of 0.7 s.
For a completely elastic material, the picture is slightly different. Breaking layers is impossible, but infinite compression is possible. Consequently, when the speed of the outer rim is close to the speed of light, for an external observer the wheel can turn into an infinitesimal point.
Rice. 6. Lorentzian deformation of a disc made of elastic material
This is if less force is required for compression than for tension. Otherwise, the shape of the wheel will remain unchanged when these forces are equal. After stopping rotation, the wheel will return to its original dimensions without any damage. In the animation, as above, you can see that the rim layers are folded in the form of an "accordion" without crossing each other. True, here it would be necessary to show a thickening of the disc in the gap between the outer rim and the axle. The disc, obviously, should take the shape of a donut when compressed. When the speed of the outer rim is reached, equal to the speed of light, the disk will be compressed into a point (or rather, into a thin tube put on the axle).
For an absolutely solid material of a wheel that does not shrink, stretch or bend, the picture will also be different from the previous ones.
Rice. 7. Lorentzian deformation of a disc made of an absolutely hard material
The outer rims cannot break and the inner rims cannot shrink. Therefore, neither the one nor the other will be destroyed, but the force of their pressure on each other will rapidly increase after the maximum rotation speed is reached. From what sources does this force arise? Obviously, due to the forces driving the wheel into rotation. Consequently, the external source will have to exert more and more effort up to infinity. It is clear that this is impossible, and we come to the conclusion: when the outer rim of an absolutely solid wheel reaches the speed √2 / 2 of the speed of light, there will be no further increase in this speed. The drive motor will hit a wall. This is about the same as running, for example, behind a tractor cart, a trailer. You can run at any speed, but upon reaching the cart, the speed will be immediately limited by its speed, the speed of the tractor.
So, let's summarize. As you can see, the behavior of the spinning wheel has strictly consistent and consistent predictions in the special theory of relativity for all variants of the wheel paradox.
The version of the Ehrenfest paradox is erroneous - the impossibility of untwisting an absolutely rigid body:
Ehrenfest's reasoning shows the impossibility of bringing an absolutely rigid body (initially at rest) into rotation
These are erroneous conclusions that do not correspond to the predictions of special relativity. In addition, in the work of Ehrenfest, which should be considered the first formulation of the paradox, there is no such reasoning. It is believed that an absolutely solid body itself is by definition impossible in special relativity, since it allows for superluminal signal transmission. Therefore, the mathematics of SRT is initially inapplicable to such bodies. Nevertheless, such a body, as we have shown, can be spun up to a speed of more than two-thirds of the speed of light. In this case, no paradoxes of SRT arise, since for an external observer there is a relativistic contraction of the whole circle, including its spokes. The statement of Ehrenfest and other authors that the spokes do not compress longitudinally is erroneous. Indeed, since the rims move without slipping relative to each other, we can glue them together, treating them as one solid disc. If now we "draw" the spokes on such a solid disc, then obviously they will decrease their length, following the decrease in the rim diameters. Also, the knitting needles can be performed as corrugation on the surface of the disc and even by making radial (or at an angle) cuts inside it. The resulting spokes and the empty spaces (space) between them move as connected parts of the rims, that is, they are objects that contract as a whole. Both the material of the spokes and the spacing between them experience tangential Lorentzian contraction in equal measure, which, accordingly, leads to the same radial contraction.
The original, widespread in the literature, author's version of the Ehrenfest paradox - the unwinding of an ordinary body - is also erroneous: the radius of the wheel is simultaneously equal to the original and shortened value.
The error lies in the statement on behalf of the theory of relativity that the radius (spoke) of the wheel does not undergo Lorentz contraction. But special relativity makes no such prediction. According to her predictions, the spokes experience the same Lorentzian contraction as the wheel rim. At the same time, depending on the material of the wheel, its part exceeding 0.7 of the radius when the rim is unrolled to light speed will either be destroyed, torn if the material is not elastic enough, or the entire wheel will experience Lorentzian compression to an infinitely small radius from the point of view of an external observer ... If you stop the wheel before its destruction and before reaching a speed of 0.7 times the speed of light, then it will take its original shape for an external observer without any damage. An elastic body, when it reaches a speed higher than 0.7 times the speed of light, may undergo some deformation. For example, if there were inclusions of brittle material in it, then they will be destroyed. After stopping the wheels, the destruction will not be restored.
Thus, it should be admitted that none of the considered formulations allows us to speak of a paradox. All kinds of the wheel paradox, Ehrenfest are imaginary, pseudo-paradoxes. Correct and consistent application of SRT mathematics allows making consistent predictions for each described situation. By paradox, we mean correct predictions that contradict each other, but this is not the case here.
After reviewing a number of sources (which, of course, cannot be called exhaustive), the following became clear. The stated solution of the Ehrenfest paradox (wheel paradox) is, apparently, the first correct solution of the paradox in the framework of the special theory of relativity since its formulation by Ehrenfest in 1909. The solution considered for the first time was discovered in October 2015 and on 10/18/2015 this article was sent for publication on the website of the International Association of Scientists, Teachers and Specialists (Russian Academy of Natural Sciences) in the section Correspondence electronic conferences.
Introduction
On the home page of one of the sites on the Internet there is an inscription: There are only about a hundred people living in the world who have understood the theory of relativity. The theory is so complex that not everyone can understand it. On the other hand, there are statements that the theory of relativity is one of the most beautiful physical theories. Apparently, all this is so. But there is a subtlety in this theory. Its mathematical apparatus, although complex, but at least in general terms is still understandable. The postulates, the initial assumptions of the theory, although original, are logically substantiated and do not contradict common sense. The conclusions of the theory, although they are often accompanied by the word "paradox", nevertheless get along well with common sense and logic. The subtlety lies in the inaccessibility of the logical substantiation of the main, cornerstone of the theory. Common sense and logic do not even allow us to simply describe the mechanics of this foundation of the theory, the mechanics of the second postulate. Neither the "paradox of twins", nor the magical transformations of Lorentz, nor the "principle of relativity", nor the "relativity of simultaneity", poorly understood by many, contradict logic and common sense and, with some effort, are understandable. But the mechanism, mechanics, the realization of the second postulate of the special theory of relativity do not even have a schematic description. In the fundamental work of Einstein "On the electrodynamics of moving bodies" (1905) this postulate (principle) is formulated as follows: "2. Each ray of light moves in a" resting "system with a certain speed V, regardless of whether this ray of light is emitted by or a moving body "
Everything seems to be simple and clear. But one has only to think about how this postulate "works", and the clarity disappears. It is known that the theory of relativity is replete with paradoxes. Let us consider some of them, how paradoxical they are, whether they contain the answer to the riddle of the mechanism of invariance of the speed of light from the second postulate of the special theory of relativity.
Chapter 1. The paradox of twins (paradox of Langevin, paradox of the clock)
In the literature, on the Internet, and in numerous forums on the Internet, there are incessant discussions and discussions on this paradox. Many of its solutions (explanations) have been proposed and continue to be proposed, from which conclusions are drawn from the infallibility of the SRT to its falsity. Einstein formulated this paradox as follows: "If at point A there are two synchronously running clocks and we move one of them along a closed curve at a constant speed until they return to A (...), then this clock upon arrival in A will lag behind in comparison with the clock, which remained motionless ... ".
At present, the wording is more common not with watches, but with twins and space flights: "If one of the twins flies off in a spaceship to the stars, then upon his return he turns out to be younger than his brother who remained on Earth" (Fig. 1). The paradox, an apparent contradiction with the theory of relativity, is that the moving twin can be considered the one that remained on Earth. Consequently, a twin flying into space should expect that the brother remaining on Earth will be younger than him.
But the paradox has a simple explanation: the two frames of reference in question are actually not equal. The twin that flew into space was not always in the inertial frame of reference during its flight.
Fig. 1. The twins paradox
At the stages of acceleration, deceleration, turn, he experienced accelerations and for this reason the provisions of the special theory of relativity are inapplicable to him at these moments. For an earthly brother, he was in motion, and his clock lagged behind, but for himself, the clock of an earthly brother went on a completely different schedule, including ahead. Therefore, there is no contradiction (paradox). Moreover, if you interpret it correctly, then in full agreement with the theory, without paradoxes and contradictions: yes, indeed, for each of the twins, the brother will be younger.
We can say that the "paradox of twins" is an ordinary phenomenon of the theory of relativity and is not in the least contradictory. This is an amazing, even amusing consequence of the theory, a consequence, mathematically rigorously described and substantiated, nothing more. It only takes a little effort to understand this beautiful math. It is surprising that there are a lot of works describing various solutions to the "paradox", but they return to it again and again, offering more and more new explanations. Is the paradox so complex that none of the solutions are final? Is it possible to conduct a real experiment to determine which of the twins "really" turns out to be younger and why? For example, what will happen if you carry out a variant of the experiment with the "paradox of twins", which can be called by analogy the "paradox of three twins." Such an experiment makes it possible to exclude the influence of non-inertial stages and more clearly see if there is a contradiction.
Let us formulate the experimental conditions in a semi-joking manner. Suppose that two planets - Earth and Yalmez, which is a colony of the Earth and located in a distant galaxy, form an inertial frame of reference. At some point in time, a spaceship flying at sublight speed is directed past the Earth towards the planet Yalmez. When a spaceship is close to Earth, an amazing event occurs: twin triplets are born. One of them was born on Earth, another on the planet Yalmez, and the third on a starship. It does not require any synchronization of clocks, scrupulous consideration of the relativity of simultaneity and other tricks of SRT in order to understand: at the moment of birth, two of the twins were at the same point in space (conventionally, of course), therefore they are of the same age. At the same time, as we can see, there are no violations of inertia: both systems - the spacecraft IFR and the Earth-Yalmez IFR without any reservations - are inertial. Therefore, we expect interesting conclusions about the age of the twins, for which it is only necessary to solve the question: how to compare their ages upon the arrival of the third twin on the planet Yalmez. Let us assume that the speed of the starship was such that the deceleration of the time rate of the moving clock is equal to two. This is approximately 0.86s kilometers per second. Let us take the distance between the Earth and Yalmez in the ISO of these planets such that the spacecraft will overcome it in 40 years, that is, L = 32.4c kilometers. After 40 years of Earth time, a twin born on a starship arrives on the planet Yalmez. The spaceship is flying by, but a dialogue takes place between twin 2 and twin 3 in one short moment.
Fig. 2. A variant of the twins paradox - three twins
In a short moment of meeting, twins 2 and 3 discovered that the second twin was older than the third. For the twin on Yalmez, this exactly corresponds to the conclusions of the theory of relativity, since in its IFR the first and second twins are always the same age. From their point of view, the third twin was moving, and his clock and age lagged behind the age of the twin on Yalmez as well as the age of the earthly twin.
How are things going from the point of view of the third twin? After all, he clearly sees: the brother is older than him, and the first is the same age as him, therefore, it turns out that there is no relativity? Could it be that the youngest one of the twins who moved on the starship turned out to be really young? When analyzing this situation, it is a weak consolation that for the third twin, the age of the first "is seen" not at all the same as the second twin sees it. But how does this happen? It’s too obvious. Moreover, it may even seem that the SRT is mistaken, because from a logical point of view and from the standpoint of common sense, we are ready to simply believe that the one flying was younger.
Chapter 2. How did all these "paradoxes" begin?
In life, as usual, everything turned out to be somewhat more complicated. The consciousness of some physicists and mathematicians refuses to accept the new views of relativity. There are many who make great efforts to refute the theory of relativity. At the same time, the deniers are trying to do this in the most exhaustive way, trying to knock out the foundation from under the theory, see, so to speak, at its very root - in mathematics. But this is an unpromising direction - the mathematics of the special theory of relativity is internally flawless, consistent, and in principle it is impossible to refute it by mathematical means.
Opponents of the special theory of relativity come up with many complex and cunning experiments, supposedly revealing contradictions in the theory of relativity. For example, one in which the beam of light is assumed to be "curved", which, of course, is not the case.
Fig. 3. Scheme from an article aimed at refuting SRT
However, a careful examination always reveals the unaccounted for features of the mathematics of the theory. As a rule, the stumbling block turns out to be the most refined phenomenon of SRT: "the relativity of simultaneity". And only a thorough, careful study of the mathematics of SRT can remove all objections, since there is not a single argument of opponents against the mathematics of SRT proper. For more than a century of the life of SRT, not a single mathematical error has been discovered in it. If the mathematics of SRT is true, then all the consequences from it are also necessarily true.
Another question is the difficulty of perceiving these conclusions and of mathematics itself. Oddly enough it sounds, but the most difficult to perceive of these conclusions are the simplest ones - the Lorentz transformations. From them follow such phenomena as contraction of a moving segment, lagging of a moving clock, relativity of simultaneity of events. These are amazing phenomena, mysterious at first glance and even paradoxical. Indeed, how does it happen that my watch lags behind yours, but yours also lags behind mine ?! This is a paradox, a contradiction! This bewilderment is directly related to the thought experiment with three twins. Let's take a closer look at the behavior of the clock next to each of the twins, denoting them with the same numbers:
Fig. 4. So whose clock is falling behind?
As mentioned above, at a fleeting meeting of the second and third twins, they found that the second twin is older than the third, and the clock of the third T3 lagged behind the clock of the second T2 (everything is exactly 20 years). Gemini 2 claims: the third flew the distance L, which took him 40 years. At the same time, as a result of the Lorentzian time dilation in the spacecraft's IFR, only 20 years have passed. Everything is correct. But the first and second twins also moved - in relation to the third. Hence, their clock must have lagged behind too - in relation to the clock of the third twin. The second twin states:
The third flew a distance L equal to 32.4 s kilometers.
No, - says the third, - I flew only 16.2s kilometers. Therefore, according to my watch, not 40 years have passed, but only 20. That is how many years I am now.
How so ?! - the second does not give up, - We measured the distance between the Earth and Yalmez, it is exactly equal to 32.4 s kilometers.
So it's the same in your ISO. And in my ISO - you are moving in relation to me, therefore this segment - the distance between the Earth and Yalmez - is shortened for me and has a length L` equal to 16.2 s kilometers.
Yes, that's right, - the second twin finally agrees, - But after all, for us, all the intervals in your ISO are shortened. Why don't you take this reduction into account?
I take into account. But now we are talking only about one segment - the interval between the planets in the Earth's ISO - Yalmez. And this segment is motionless in relation to the Earth, and moves in relation to me. Therefore, it is reduced. So it turns out that I flew the distance from the point of view of my ISO, exactly two times less than measured in your ISO. And since the first brother was moving in relation to me at a speed of 0.86s kilometers per second, then his clock lagged behind in relation to mine. Therefore, he is now only 10 years old. After all, I am already 20 years old.
What are you talking about? - exclaimed the second brother in surprise, - He is exactly the same as me! We are the same age and our age is the same.
Exactly, - summed up the third brother, - You correctly noticed: you are the same age, since you are in the same ISO. It's the same age for each other. But for me, out of my ISO, the age of the first brother is 10 years, this is precisely what relativity consists in: the values of quantities are determined by the frame of reference they are obtained from.
To this conversation of the twins, we can add that he exactly describes the actually observed physical process with the life time of pions, in which the peony acts as a third twin and, due to the movement, "lives" longer than its "immobile" (earthly) counterparts, which gives him the opportunity fly through the Earth's atmosphere much further than is possible with its "standard lifespan".
Discussions on the fallacy of SRT are not uncommon on forums devoted to the theory of relativity. But their main misfortune and fallacy, unfortunately, is their unwillingness to delve into the mathematics of the theory. The conclusions of the theory contradict only the usual, classical ideas. These familiar notions are applied to theory contrary to its claims. The theory of relativity is accused of false statements that it did not make! She is credited with conclusions that she does not draw, and then they try to refute them. It is not easy to understand how it turns out that segment A is shorter than segment B, but segment B is shorter than segment A. Or T1 is lagging behind T2, but at the same time T2 is lagging behind T1. Of course, such a comparison is meaningless, two quantities cannot be less than each other at the same time. The trick is that when you have two clocks, there are actually four quantities to compare:
1. Readings of watch A from the point of view of A;
2. Readings of the clock A from the point of view of B;
3. Readings of the clock B from the point of view of A;
4. Readings of clock B from the point of view of B.
The fact that the readings of item 1 are greater than the readings of item 3 does not in any way contradict the fact that the readings of item 2 are less than the readings of item 4. But to understand this, it is necessary to carefully read the provisions of the SRT, in particular with its fundamental principle of relativity. Only in this case it will become clear how to compare the readings of the clocks in clause 1 - clause 4. The same can be said about the lengths of the segments, which also have not two meanings, but four. Thus, the Lorentz phenomena do not give any grounds for accusations of the SRT in paradox and contradiction to common sense.
Chapter 3. What Michelson's Experience Refuted
As we have shown above, the most common ideas about SRT do not contain any contradictions or paradoxes in the deep sense of the word. At the level of conclusions, everything is quite simple and consistent. It may seem that, in general, "SRT is elementary!" Why, then, does the controversy around her not subside? Why do many physicists and mathematicians try to find contradictions in it, reject it? Is there any secret of the SRT that is stated in the title of this article?
Many experiments have been and are being carried out in the world, aiming to find not just another proof of the theory's validity, but at least something that does not agree with it. But all is in vain - the service station receives only one more confirmation.
A few years before the advent of the theory of relativity, in 1881, Michelson conducted an experiment that could well become the parent of the theory of relativity and Lorentz transformations. The main goal of the experiment was the search for an absolute reference system associated with the ether. At the dawn of its origin, SRT, referring to this experience, directly rejected such a frame of reference. Michelson's experiment, in fact, did not show the presence of such a system, the presence of ether, and was a confirmation of the provisions of the theory of relativity.
According to the theory of the stationary ether that existed at that time, it was possible to measure the absolute motion of the Earth in relation to the ether. Let's draw the following analogy. Three arrows are aimed at the target (Fig. 5). The first shooter is on the platform that approaches the target. The second is motionless. The third is on a platform that moves away from the target. The moment all three arrows were level, they fired. The first to reach the target is the bullet fired by the first shooter; then the second shooter's bullet will reach the target; the third shooter's bullet will hit the target last. The time difference between bullets hitting the targets depends on the speed of the platforms. That is, by measuring the delay of the bullet hitting the target, we can estimate the speed of the platform (analogue of the Earth) movement:
Fig. 5. Three arrows and targets
By analogy with arrows, the idea appeared to determine the absolute speed of the Earth. But it turned out that the situation with the photon is different. If the arrows shoot light beams at the target, then they will all reach the target at the same time, regardless of the speeds of the platforms. Despite the fact that the IFR has acquired speed, the speed of the photon remains the same and still t = L / c. This is rather strange, so let's analyze the process. Without going into the technical details of the experiment and Michelson's installation, let us consider the physical essence of the experiment using the Michelson technique. To do this, take a platform of length L, which is crossed by a photon emitted by an unknown source and simply passing by. A photon for observers on the platform will fly through it in time t = L / s. Then we will accelerate the platform to the speed v and again measure the time of flight of the photon. The time will be exactly the same. But why? The platform is overclocked, and the photon, as if nothing had happened, overcomes it in the same time. Let's conduct a mental experiment on a setup similar to the Michelson setup shown in Fig. 6. Let's depict the photon as a baseball, and the mirror as a bat, which reflects the photon-ball from the opposite wall and returns it to the target. If the observer knows nothing about the motion of his frame of reference, he considers it to be at rest and calculates that the photon will overcome the platform in time t = 2L / c (the way there and the way back).
Fig. 6. The flight of a photon from the point of view of an observer inside the IFR
However, an outside observer sees that the platform is moving. He also sees: the light in one case catches up with the mirror at the opposite end of the platform, and in the other it flies towards the target (Fig. 7). But this is seen only by an observer who remained motionless after the acceleration of the platform, that is, an observer conditionally associated with the propagation medium, with the ether (as suggested by Lorenz and Michelson).
Fig. 7. Photon flight from the point of view of an external observer
It can be seen in Fig. 7 that for an external observer, the time of movement of a photon along the platform back and forth will be:
Here we marked the time and length of the platform with shaded values. First, we are not sure that this time t` is exactly equal to the time on a stationary platform; secondly, we suspect (like Michelson) that the moving platform should be reduced in size, since the time of crossing the platform for the observer on the platform has not changed, but the platform is moving, and the path for light has clearly changed, presumably more. On the other hand, if the path for light has changed, and the speed, as shown by measurements, remains the same, then the travel time for the photon has also changed. Apparently, it changed in the same direction as the length of the platform - it decreased, and exactly as much as the platform decreased, because these three quantities are related by the formula: t = L / c. There is one more obvious circumstance that we can verify experimentally: in a moving IFR (platform), the speed of light is the same when moving back and forth. Therefore, in equation (1), we put the same speed of the photon everywhere. Let's transform the equation:
The resulting equations do not tell us anything yet. Let's try to compare the obtained values. It is interesting to know how the time has changed in the flight of a photon through a moving platform. Let's calculate the ratio:
The asymmetry of the equation is striking. Let's try to fix it at random and restore symmetry, intuitively expecting interesting conclusions:
An elementary analysis of the obtained equality suggests the following conclusion:
The time t is as many times less than the time t`, how many times the length L is greater than the length L`. And what are these values? Above, we assumed that in a moving platform, time slowed down, and its length changed (decreased). At the same time, we assumed that these two changes are equal: how much the time decreased, the length also decreased by the same amount. Let's see if this corresponds to equality (7). Time t is the time of flight of a photon through the platform for an observer on this platform, and L is the length of the platform for this observer. Obviously, the observer did not notice anything during the acceleration of the platform, nothing happened to him, he, generally speaking, might not have known that the platform was moving. Therefore, these two values are initial, not reduced, those that were known before the start of the experiment. And what are the quantities t` and L`? Based on the fact that after overclocking the platform, the results of Michelson's experiment remained the same, we concluded that the platform shrank, and the time in it slowed down. But who is observing the contraction of the platform and the contraction of the pace of its clock? Obviously, this is an observer who sees the movement of the platform - motionless, remaining in the ether frame. Therefore, he sees a platform of length L` and the time t`, during which the photon flew through the platform back and forth. We know that on the platform the clock began to run slower, that is, the time t elapsed on the platform is less than the time elapsed in the stationary frame of reference t '.
Similarly, we conclude: in a fixed IF, the length of the platform is seen to be shortened to the value L`, against the original length L. Above, we came to the conclusion that the decrease in time should be exactly equal to the reduction of the platform, that is:
From where, after the transformations, we find:
From equation (8) we find the same expression for time:
Here the observant reader will notice the same contradiction that Akimov apparently discovered and called it the "stroke paradox." In our case, we ourselves have chosen the designations of the times. What to call "internal time of ISO" is quite arbitrary. We have dashed the time that is included in the equation containing the dashed platform length. It would seem logical. However, the shaded length of the platform is its shortened length, that is, the length of the "moving rod", which, according to the SRT rules, is indeed marked with a stroke. The time we "shaded" is that which is measured by the clock in the motionless IFR, which already contradicts the rules of the SRT. Therefore, in the last equation (10), it would be more correct to rearrange the prime to the left side. So, we found that the technique of Michelson's experiment easily and unambiguously leads to one of the conclusions from the Lorentz transformations. At the same time, we did not need to use the concept (substance) of the ether and the absolute frame of reference, mentioning them only as a tribute to tradition. Consequently, this experiment does not contain logical contradictions or assumptions that contradict common sense, or at least cause difficulties in understanding them.
Chapter 4. The Great Mystery
It turns out that the special theory of relativity is a harmonious, complete system, in which there are no questions requiring solution, consideration, comprehension? No, it’s not like that. There is still at least one white spot in it. Common sense and elementary logic cannot accept the basic principle of the special theory of relativity, which is the cause of all the consequences of SRT (Lorentz transformations, relativity of simultaneity). Neither SRT itself, nor physics, nor mathematics give any description of the mechanism of action of the second principle (postulate) of SRT. How does the phenomenon occur that the speed of light does not depend on the speed of the source? The manifestations of the principle are logical, but the principle itself is not.
Let's return to the Michelson platform (Fig. 8). Let's disperse it. It is either declining or not. It depends only on an outside observer, since it is for him that it changes its size. But we are not referring to the opinion of an outside observer. We have experienced acceleration during acceleration and we know for sure that the platform speed has changed. Michelson's experiment showed no change in the interference pattern. Has the platform shrunk? There is no external observer, Michelson's experience is. Who has the platform shrunk for? Absolutely? But this is unacceptable for the service station. For the platform itself, there can be no real reduction. Regardless of the movement, it cannot contract. But the interference picture has not changed! The speed of light during the movement of the platform remains the same.
Why the speed of light is constant is easy to imagine. For example, this is a property of matter (analogue of the ether), that is, the fundamental principle that forms everything that exists: matter, physical vacuum, fields, and so on. This fundamental principle may possess some inertia in the transmission of its deformations, which manifest themselves as the movement of matter, radiation, and fields. This explanation is in excellent agreement with the second postulate of the STR: the emitted photon subsequently interacts only with the medium, which prevents it from accelerating above the speed of light. But this does not explain the constancy of the speed in the general case, in all IFRs. If the platform is moving, then for the speed of light to remain constant in it, the platform must contract absolutely.
Fig. 8. Absolute reduction of the moving platform
We will take a certain state of the platform as the initial one and consider it a state of rest, the absence of movement of the platform (Fig. 8), that is, v = 0. A photon is moving along this platform at the speed of light, shown in the figure as a green baseball. The length of the platform for observers who are on it is L. The time it takes for a photon to fly by is equal to L / s. There are no external observers, and there is no one from outside to draw conclusions about the size of the platform and the pace of the clock on it.
Now we accelerate the platform to speed v. The fact that the platform speed is increasing, we can objectively judge by the acceleration. Using Newton's formulas and the duration of the accelerating force, we can estimate the speed of the platform. We are not interested in the exact value of this speed, but we can measure it by the speed of removal of the beacon left outside the platform. We repeat the experiment with the flight of a photon through the platform. The time of this flight is still L / s. But the photon has nothing to do with the platform, so one would expect that its flight time should increase:
These assumptions do not imply the presence of external observers, that is, the absolute shortening of its length and the slowing down of the pace of time are clearly recorded on the platform. This is inevitable, since we know for sure that the platform has increased in speed. But at the same time, we have no way to determine either the reduction in the length of the platform, or the deceleration of the pace of time, since all the reference rulers on the platform decrease to the same extent and the pace of the course of all reference clocks on it slows down.
Such an explanation of the invariance of the speed of light would be quite suitable if there were no external observers who did not subject their platform to acceleration. On the one hand, they would also record the change in the length of the platform under consideration to L`, and the rate of time to t`. But on the other hand, all the same changes in lengths and times should also occur for outside observers, since the principle of relativity allows us to consider them as moving, and the platform under consideration at rest. Otherwise, for a moving platform, time on a stationary platform will be accelerated, and the segments will be elongated.
Hence the conclusion inevitably follows: an overclocked platform cannot change its length absolutely. This contradicts the previous conclusion.
Let us once again consider equations (11) and (12) as the results of observation from the side of an external, conventionally stationary platform. In this case, everything converges perfectly and agrees with the principle of relativity. These two equations apply to an outside observer, no matter what platform he is on. In this case, the speed v is the speed of the relative movement of the two platforms. Each of the observers sees that the platform moving past him is contracting, and the pace of time on it slows down. However, in this case, the question of the invariance of the speed of light arises again: how did it happen that after an explicit, experimentally recorded increase in the speed of the platform, the time of flight of a photon through it remained the same as before acceleration? We can no longer make assumptions about the absolute reduction in the length of the platform and the slowing down of the pace of the clock on it. The relative reduction makes sense only for an outside observer, for observers on the platform itself there can be no "relative to themselves". But when it moves, it runs away from the light, so the light must move faster! And only for observers on this platform, since for everyone else it moves at the same speed as before acceleration.
And what did we get? It turned out that the platform cannot shrink, but it cannot not shrink either. That is, it was not possible to explain the constancy of the speed of light in a stationary / moving platform. This is the Great Secret of the Special Theory of Relativity: the mechanism of maintaining the speed of light in a stationary / moving IFR cannot be described logically, without contradicting common sense. There is no such mechanism in SRT: not only the reason is not clear, but even the very elementary external description, how does the speed of light manage to remain unchanged during the acceleration of the system? How to describe the invariance of the speed of light? What does it look like? Such is the simple question: HOW? The explanation from the category "due to the curvature of space-time" does not explain anything, but begs for Occam's razor and Feynman's "nuka-tuki".
Chapter 5. Theory of relativity versus quantum mechanics
In addition to the Great Mystery, SRT has another controversial issue - the conflict with quantum mechanics. Moreover, the conflict is also logically inexplicable and does not fit into the framework of common sense.
When discussing the phenomenon of entanglement of particles in quantum mechanics and the instantaneousness of the collapse of the wave function, the absence of a contradiction between quantum mechanics and the special theory of relativity is always emphasized. However, the phenomenon of entanglement, nevertheless, allows, in principle, to organize an experiment, which can clearly show that clocks moving relative to each other run synchronously (Fig. 9). This means that the SRT statement that the moving clock lags behind is erroneous. There is good reason to believe that there is an unavoidable contradiction between quantum theory and special relativity regarding the rate of transmission of interactions and quantum nonlocality. The position of the quantum theory about the instantaneousness of the collapse of the state vector contradicts the SR postulate about the limited rate of transmission of interaction, since there is a way to use the collapse to generate a synchronization signal, which is actually an information signal that instantly propagates in space. Hence, it follows that one of the theories is quantum or special theory of relativity, or both theories require revision in the question of the rate of transfer of interaction. For quantum theory, this is the rejection of the quantum correlation of entangled particles (nonlocality) with the instantaneousness of the collapse of the wave function at any distance; for SRT, this is the limit of the interaction transfer rate.
The essence of quantum synchronization is as follows.
Fig. 9. Quantum clock sync
Two entangled particles (photons) instantly acquire their own states when the common wave function collapses - this is the position of quantum mechanics. Since there is at least one IFR in which each of the photons receives its own state within the measuring device (target), there is no reasonable reason to assert that there are other IFRs in which these states were received by the photons outside the measuring devices. Hence the inevitable conclusion that the triggering of two meters occurs simultaneously from the point of view of any IFR, since for any IFR both meters (targets in Fig. 9) worked simultaneously due to the collapse of the wave function. In particular, this means that the own meter of the stationary IFR worked absolutely simultaneously with the meter in the moving IFR, since the entangled quantum particles (photons) at the moment of collapse were within the measuring devices, and the collapse occurs instantly. The use of signatures (sequences of meter signals) allows you to subsequently show the synchronization of the clock (clocks T1 and T2).
Chapter 6. Superluminal speed of light
Corpuscular wave dualism emerged as a compromise between the two manifestations of the essence of the photon (and other quantum particles). Two forms of manifestation are assigned to quantum particles: a wave and a corpuscle. In this case, the wave is characterized by a clearly defined length. For example, this is clearly taken into account in the standard meter, which is defined as a given number of periods of some radiation. The wave behavior of a photon is used to explain the cosmological redshift, the Doppler effect. That is, the wave manifestation of a photon is characterized by its spatial extent. This is not a point substance, it is some kind of spatially distributed formation. Considering the speed of its propagation, the photon has a rather extended form. Let's depict a photon in the form of such a kind of "spear":
Fig.10 Photon as a "spear"
But when interacting with other particles and with matter, the photon manifests itself as a particle, as if a "drop of energy", which, in all likelihood, has a small length. Otherwise, upon contact with the object, the "photon head" enters into interaction earlier than its "tail". Consequently, either the photon interacts smoothly, as if overflowing from one container to another, or collapses instantly into a point. In the latter case, the speed of its "tail" must be higher than the speed of light.
The phenomenon of interference also testifies to the superluminal ability of a photon to "collapse" at the point of interaction. When a photon passes through a semitransparent mirror (splitter), it appears to be simultaneously in two separated points in space (Fig. 11), which can be at a fairly large distance from each other. The photon can be fixed (registered, measured) in each of the channels, which suggests that it will indeed split into two halves. However, these two halves have a unique property: they collapse into one another and only one into the other.
Fig. 11. Collapse of a halved photon
Moreover, no obstacle can prevent this collapse: neither fields, nor matter, nor distance. This assumption is supported by the phenomenon of entanglement of quantum particles, which "feel" each other instantly at any distance. True, the distance may actually be limited and somehow depend on the Heisenberg uncertainty principle. Literature:
Date of access to all URLs 12.05.2012
1. Putenikhin P.V., The main mystery of quantum physics, 2009,
http://econf.rae.ru/article/6357
http://sciteclibrary.ru/rus/catalog/pages/9818.html
http://quantmagic.narod.ru/volumes/VOL642009/p4126.html
http://www.scorcher.ru/theory_publisher/show_art.php?id=363&editing=1
4. Putenikhin PV, Contradiction between quantum mechanics and STR, 2010,
http://econf.rae.ru/article/6360
http://econf.rae.ru/pdf/2011/11/714.pdf
http://www.sciteclibrary.ru/rus/catalog/pages/10373.html
http://quantmagic.narod.ru/volumes/VOL732010/p3115.html