Physical properties of natural gas. Natural gas composition
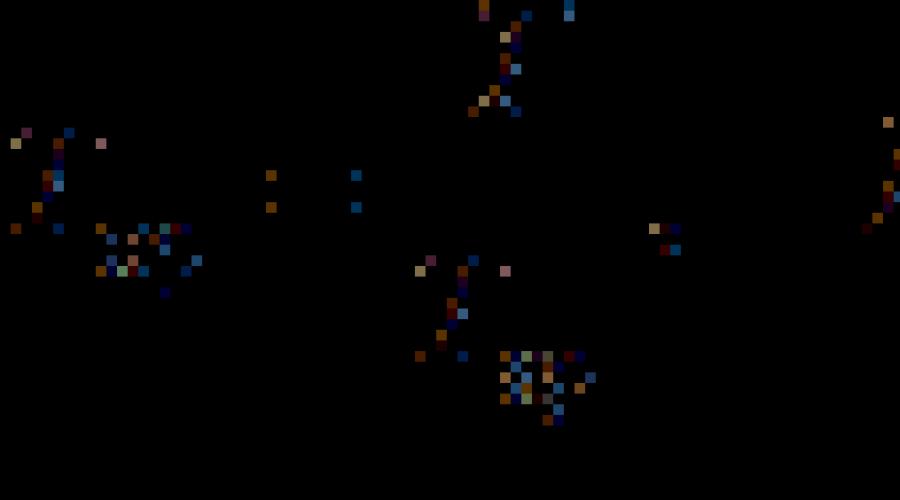
Physical and chemical properties of natural gases. Calculation of the gas mixture.
Gases are divided into natural and artificial. Currently, natural gases are mainly used for gas supply. They have a complex multicomponent composition. Natural gases are classified into three groups depending on their origin:
1. Gases, extracted from purely gas fields, consist of methane by 82. ..98%;
2. Gases from gas condensate fields containing 80 ... 95% methane;
3. Gases of oil fields (associated petroleum gases) containing 30 ... 70% methane and a significant amount of heavy hydrocarbons. Gases with a content of heavy hydrocarbons (from propane and above) less than 50 g / m 3 are usually called dry or "lean", and with a high content of hydrocarbons - "fat".
Recently, there has been a lot of talk about the fourth group of natural gases - shale gas and coalbed methane. Shale gas is a natural gas produced from shale and consists primarily of methane. Shale gas is generated by the degradation of kerogen contained in oil shale; the gas is there in microcracks. Large-scale commercial production of shale gas began in the United States in the early 2000s at the Barnett Shale field. Thanks to a sharp increase in its production, called in the media a "gas revolution", in 2009 the United States became the world leader in gas production, and more than 40% came from unconventional sources (coalbed methane and shale gas). Coal bed methane is contained in coal-bearing sediments. Causes explosions in coal mines. Coal bed methane is a cleaner and more efficient fuel than coal.
Natural gases are colorless, odorless, and in a normal state, they are in different states of aggregation. Methane, ethane and ethylene-gaseous, propane, butane, butylene and propylene - in the form of liquid vapors, and under high pressures - liquid substances. Heavy hydrocarbons, starting with isopentane in their normal state, are liquids, they are part of the gasoline fraction. In order for natural gases to smell for safety reasons, special substances are specially added to them - odorants.
Usually, gases are considered under two conditions:
1. Normal condition - R n = 0.1013 MPa (normal atmospheric pressure), T n = 273.16K (0 0 C);
2. The standard condition is R st = 0.1013 MPa (normal atmospheric pressure), T st = 293.16K (20 0 C - room temperature).
To perform the hydraulic and thermal calculation of gas pipelines and to calculate the operating modes of compressor stations, it is necessary to know the basic properties of natural gases: density, viscosity, gas constant, pseudocritical values of temperature and pressure, heat capacity, thermal conductivity coefficient, compressibility and Joule - Thomson coefficients.
Molar mass of gas ( M), it is the mass of 1 mole of gas. One mole of a substance is approximately 6 billion trillion. the number of any molecules (equal to Avogadro's number: N A = 6.02 10 23). Its dimension [ M] = kg / mol, or [ M] = g / mol. The molar mass of a gas is found in terms of its molecular mass. For example, the molecular mass of hydrogen is approximately 2, then its molar mass is M≈2g / mol = 2 · 10 -3 kg / mol. For oxygen M≈32g / mol, for nitrogen M≈28g / mol, for propane (C 3 H 8) M≈12 3 + 1 8 = 44g / mol, etc. The density of a gas is the mass of a unit volume:
The relative density of gas in air Δ is the ratio of the density of the gas to the density of the air. For all states of the gas, the following expression takes place:
here [ M] = g / mol, 28.96 g / mol is the molar mass of air. For standard condition
here ρ is the gas density at standard conditions (air density at standard conditions is 1.205 kg / m 3, for normal conditions 1.29 kg / m 3).
Any gas in an amount of 1 mol in a normal state takes up a volume of about 22.4 · 10 -3 m3, therefore the density of the gas under normal conditions
Here [ M] = g / mol, but this expression is incorrect for the standard state.
Viscosity (dynamic) of gas μ , a [ μ ] = Pa · s. The viscosity of a gas is determined by the transfer of momentum (from one layer to another) by a gas molecule during its transition from one flow layer to another. Therefore, the viscosity of the gas strongly depends on temperature and is almost independent of the gas pressure (up to 4 MPa). Dynamic μ and kinematic ν gas viscosity of gas is related by the ratio:
Specific heat of gas at constant pressure with, a [ with] = J / (kg · K). It is equal to the amount of heat required to heat 1 kg of gas by 1K at constant pressure. Gas pressure R shows the force acting normal to the unit area of the vessel wall from the side of gas molecules. [ R] = atm, [ R] = Pa, or [ M] = MPa. 1 MPa = 10 6 Pa≈10 Atm. The gas temperature is determined on the Kelvin and Celsius scales, they are related by the ratios:
In many cases, the gas can be converted to a liquid by compression. However, the gas temperature must be below the critical ( T cr). If it is equal to or higher than the critical temperature, then at no pressure does the gas turn into liquid. And also, if the gas pressure is equal to or higher than the critical pressure ( R cr), then in the future, at no temperature, the gas does not turn into a liquid.
The main types of gas transportation include rail transport, sea transport and pipeline transport. Each mode of transport has a strength and a weakness.
To calculate the gas mixture, you need to know the equation of state of the gas. The gas equation of state connects the basic parameters of a gas, such as its quantity, volume, pressure and temperature. From the school and higher course of physics, you know the equations of state of Mendeleev-Clapeyron, Van der Waals, and for gas pipelines it is convenient to have the equation of state of a gas written in terms of the compressibility of a gas:
where R- gas constant, determined for a specific gas, or gas mixture. It is found through the universal gas constant (8.314 J / (mol K)):
units of measurement in expression (8): [ m] = kg, [ M] = kg / mol, ([ R] = Pa). z in expression (128) is called the gas compressibility (coefficient of compressibility) for a particular gas, or gas mixture. The compressibility factor depends on the state of the gas. It is usually determined by special nomograms depending on the reduced temperatures and pressures, or in an analytical form using a formula recommended by industry design codes. The quantities are called reduced gas parameters:
. (129)
The compressibility factor takes into account the deviation of the properties of natural gas from the laws of an ideal gas. There are 2 formulas recommended by the industry design code for the compressibility factor. But both of them are approximate and give almost the same results with the real parameters of the main gas pipeline. The first of the formulas:
And the other formula is:
. (131)
In these formulas for the main gas pipeline, the average values of pressure and temperature are taken:
. (132)
The first formula is convenient for the calculation.
Usually the amount of a gas mixture (or gas) is conveyed through its volume. But the volume depends on the real state of the gas, that is, if the working volume of the gas is known for a given state V, then in other states the corresponding volumes of gas will be different. For clarity, volumes are taken for normal and standard conditions. In technical calculations, and in calculations for storage and transportation of gas, as well as in commercial calculations, the volume of gas is reduced to a standard condition.
The formula for bringing the working volume of gas to the normal condition (normal volume) is as follows:
. (133)
The formula for bringing the working volume of gas to the standard condition (commercial volume):
. (134)
here [ R] = MPa.
The required physical and chemical properties of the gas mixture include the following parameters: molar mass M, pseudocritical temperature T cr, pseudocritical pressure R cr, pseudo-critical volume V cr, specific heat of gas at constant pressure, dynamic viscosity and coefficient of thermal conductivity λ ... They are determined through the properties of each component of the mixture.
The composition of the gas mixture is characterized by mass, or volume, or molar fractions of each component. The volume fractions of each component of the mixture are equal to the corresponding molar fractions and it is easier to calculate with them. Let the volume fractions of each component of the mixture at 1 , at 2 , at 3, etc. Then the following formula is always valid for the entire gas mixture:
The rest of the parameters of the mixture in different sources are determined in different ways. The easiest way is to determine by the additivity rule (proportional addition). This method is easy to use, but not very accurate. It is used for rough calculations and gives a very good result when the proportion of methane in the mixture is at least 96% (especially when calculating the viscosity). So.
Characterization of methane
§ Colorless;
§ Non-toxic (non-toxic);
§ Odorless and tasteless.
§ Methane contains 75% carbon, 25% hydrogen.
§ The specific gravity is 0.717kg / m 3 (2 times lighter than air).
§ Ignition temperature Is the minimum initial temperature at which combustion begins. For methane, it is equal to 645 o.
§ Combustion temperature Is the maximum temperature that can be reached with complete combustion of the gas, if the amount of air required for combustion exactly matches the chemical formulas of combustion. For methane, it is equal to 1100-1400 о and depends on the combustion conditions.
§ Heat of combustion Is the amount of heat that is released during the complete combustion of 1 m 3 of gas and it is equal to 8500 kcal / m 3.
§ Flame propagation speed equal to 0.67 m / s.
Air-gas mixture
In which gas is located:
Up to 5% does not burn;
5 to 15% explodes;
Over 15% burns when additional air is supplied (it all depends on the ratio of the volume of gas in the air and is called explosive limits)
Combustible gases are odorless, for their timely detection in the air, quick and accurate detection of leaks, the gas is odorized, i.e. give a smell. To do this, use ETHYLMERKOPTAN. Odorization rate 16 g per 1000 m 3. If there is 1% natural gas in the air, you should smell it.
The gas used as a fuel must comply with the requirements of GOST and contain harmful impurities per 100m 3 no more:
Hydrogen sulfide 0.0 2 G / cubic meter
Ammonia 2 gr.
Hydrocyanic acid 5 gr.
Resin and dust 0.001 g / m3
Naphthalene 10 gr.
Oxygen 1%.
The use of natural gas has several advantages:
· Absence of ash and dust and removal of solid particles into the atmosphere;
· High heat of combustion;
· Ease of transportation and incineration;
· Easier work of service personnel;
· Improved sanitary and hygienic conditions in boiler houses and adjacent areas;
· Wide range of automatic regulation.
When using natural gas, special precautions are required because possible leakage through leaks at the joints of the gas pipeline and fittings. The presence of more than 20% of the gas in the room causes suffocation, its accumulation in a closed volume over 5% to 15% leads to an explosion of the gas-air mixture. With incomplete combustion, carbon monoxide is emitted, which, even at a low concentration (0.15%), is poisonous.
Combustion of natural gas
By burning is called a fast chemical combination of combustible parts of fuel with oxygen in the air, occurs at high temperatures, accompanied by the release of heat with the formation of flame and combustion products. Burning happens complete and incomplete.
Complete burning- occurs when there is a sufficient amount of oxygen. Lack of oxygen causes incomplete combustion, in which less heat is emitted than in full, carbon monoxide (poisonous effect on the operating personnel), soot is formed on the surface of the boiler and heat losses increase, which leads to excessive consumption of fuel, a decrease in the efficiency of the boiler, and pollution of the atmosphere.
Natural gas combustion products are- carbon dioxide, water vapor, some excess oxygen and nitrogen. Excess oxygen is contained in combustion products only in those cases when combustion occurs with excess air, and nitrogen is always contained in combustion products, because is an integral part of air and does not take part in combustion.
The products of incomplete combustion of gas can be carbon monoxide, unburned hydrogen and methane, heavy hydrocarbons, soot.
Methane reaction:
CH 4 + 2O 2 = CO 2 + 2H 2 O
According to the formula for the combustion of 1 m 3 of methane, 10 m 3 of air is needed, in which there is 2 m 3 of oxygen. In practice, for the combustion of 1 m 3 of methane, more air is needed, taking into account all possible losses, for this the coefficient is applied TO excess air, which = 1.05-1.1.
Theoretical air volume = 10 m 3
Practical air volume = 10 * 1.05 = 10.5 or 10 * 1.1 = 11
Completeness of combustion fuel can be determined visually by the color and nature of the flame, as well as using a gas analyzer.
Transparent blue flame - complete combustion of gas;
Red or yellow with smoky streaks - incomplete combustion.
Combustion is regulated by increasing the air supply to the furnace or decreasing the gas supply. This process uses primary and secondary air.
Secondary air- 40-50% (mixed with gas in the boiler furnace during combustion)
Primary air- 50-60% (mixed with gas in the burner before combustion) a gas-air mixture is used for combustion
Combustion characterizes flame spreading speed Is the speed at which the flame front element distributed by a relatively fresh jet of gas-air mixture.
The rate of combustion and flame spread depends on:
· From the composition of the mixture;
· From temperature;
· From pressure;
· On the ratio of gas and air.
The burning rate determines one of the main conditions for the reliable operation of the boiler house and characterizes it flame separation and breakthrough.
Flame separation- occurs if the speed of the gas-air mixture at the outlet of the burner is greater than the burning speed.
The reasons for the separation: excessive increase in gas supply or excessive vacuum in the furnace (draft). Flame separation is observed during ignition and when the burners are turned on. The separation of the flame leads to gas contamination of the furnace and boiler gas ducts and to an explosion.
Flame breakthrough- occurs if the speed of flame propagation (burning speed) is greater than the speed of the outflow of the gas-air mixture from the burner. The breakthrough is accompanied by combustion of the gas-air mixture inside the burner, the burner gets hot and fails. Sometimes the breakthrough is accompanied by a pop or explosion inside the burner. In this case, not only the burner can be destroyed, but also the front wall of the boiler. Breakthrough occurs with a sharp decrease in gas supply.
If the flame breaks off and breaks through, the maintenance personnel must stop the fuel supply, find out and eliminate the cause, ventilate the furnace and gas ducts for 10-15 minutes and re-ignite the fire.
The combustion process of gaseous fuel can be divided into 4 stages:
1. The outflow of gas from the nozzle of the burner into the burner under pressure at an increased speed.
2. Formation of a gas-air mixture.
3. Ignition of the resulting combustible mixture.
4. Combustion of a combustible mixture.
Gas pipelines
Gas is supplied to the consumer through gas pipelines - external and internal- to gas distribution stations located outside the city, and from them through gas pipelines to gas control points Hydraulic fracturing or gas control device GRU industrial enterprises.
Gas pipelines are:
· high pressure of the first category over 0.6 MPa up to 1.2 MPa inclusive;
· high pressure of the second category over 0.3 MPa to 0.6 MPa;
· medium pressure of the third category over 0.005 MPa to 0.3 MPa;
· low pressure of the fourth category up to 0.005MPa inclusive.
MPa - means Mega Pascal
Only medium and low pressure gas pipelines are laid in the boiler room. The section from the distribution gas pipeline of the network (city) to the room, together with the disconnecting device, is called input.
An inlet gas pipeline is considered to be a section from a disconnecting device at the inlet, if it is installed outside the room to an internal gas pipeline.
There should be a valve at the gas inlet to the boiler room in an illuminated and convenient place for maintenance. There must be an insulating flange in front of the valve to protect against stray currents. At each branch from the distribution gas pipeline to the boiler, at least 2 disconnecting devices are provided, one of which is installed directly in front of the burner. In addition to fittings and instrumentation on the gas pipeline, an automatic device must be installed in front of each boiler to ensure the safe operation of the boiler. To prevent the ingress of gases into the boiler furnace, in the event of faulty shutdown devices, purge plugs and safety gas pipelines with shutdown devices are required, which must be open when the boilers are inactive. Low-pressure gas pipelines are painted yellow in boiler rooms, and medium-pressure gas pipelines are painted yellow with red rings.
Gas-burners
Gas-burners- a gas burner device designed to supply a prepared gas-air mixture or separated gas and air to the combustion site, depending on the technological requirements, as well as to ensure stable combustion of gaseous fuel and control the combustion process.
The following requirements are imposed on burners:
· The main types of burners must be serially produced at factories;
· Burners must ensure the passage of a given amount of gas and the completeness of its combustion;
· Ensure the minimum amount of harmful emissions into the atmosphere;
· Must work without noise, separation and flame breakthrough;
· Should be easy to maintain, convenient for revision and repair;
· If necessary could be used for reserve fuel;
· Samples of newly created and operating burners are subject to GOST testing;
The main characteristic of burners is its thermal power, which is understood as the amount of heat that can be released during the complete combustion of the fuel supplied through the burner. All these characteristics can be found in the burner data sheet.
Approximate Physical Characteristics (composition dependent; under normal conditions, unless otherwise noted):
Density:
· From 0.68 to 0.85 kg / m³ (dry gaseous);
· 400 kg / m³ (liquid).
· Auto-ignition temperature: 650 ° C;
· Explosive concentrations of a mixture of gas with air from 5% to 15% by volume;
· Specific heat of combustion: 28-46 MJ / m³ (6.7-11.0 Mcal / m³) (ie, this is 8-12 kWh / m³);
· Octane number when used in internal combustion engines: 120-130.
· 1.8 times lighter than air, so when leaking it does not collect in the lowlands, but rises up [
Chemical composition
The main part of natural gas is methane (CH 4) - from 92 to 98%. Natural gas can also contain heavier hydrocarbons - methane homologues:
Ethane (C 2 H 6),
Propane (C 3 H 8),
Butane (C 4 H 10).
as well as other non-hydrocarbon substances:
Hydrogen (H 2),
Hydrogen sulfide (H 2 S),
Carbon dioxide (CO 2),
Nitrogen (N 2),
· Helium (Not).
Pure natural gas is colorless and odorless. To facilitate the possibility of detecting a gas leak, odorants are added to it in a small amount - substances that have a pungent unpleasant odor (rotten cabbage, rotten hay, rotten eggs). The most commonly used odorant is thiols, for example ethyl mercaptan (16 g per 1000 m³ natural gas).
[kg · m -3]; [m 3 · kg -1] - specific volume.
F (P, v, T) = 0 is the equation of state of the gas.
Natural gas composition:
4. Isobutane
5.n Bhutan
6.n Pentane
µ - molecular weight
ρ - normal density
- gas density in air
P cr - critical pressure
T cr - critical temperature.
Natural gas equation of state; features of gas isotherms. Critical situation. The critical state of methane and its homologues. Liquefaction of gases.
- the equation of state of the gas.
With an increase in pressure and a decrease in temperature, the gas turns into a liquid state.
Perfect gas. Clapeyron-Mendeleev equation. Real gas. Compressibility. Supercompressibility coefficient. The given parameters. Formula for calculating the supercompressibility coefficient.
,
- the equation of state of a perfect gas.
R 0 = 8314
for real gas:
,
z is the compressibility factor.
Gas equation of state.
Gas equation of state- functional relationship between pressure, specific volume and temperature, which exists for all gases in a state of thermodynamic equilibrium, that is .
Graphically, this relationship is depicted by a family of isotherms.
At a temperature higher than the critical temperature, the gas always remains in a gaseous state at any pressure. At a temperature lower than the critical one, during gas compression, if a certain specific volume is reached, gas condensation begins, and it passes into a two-phase state. When a certain specific volume is reached, the gas condensation stops and it acquires the properties of a liquid.
The equation of state for an ideal gas is described by the Mendeleev-Clapeyron equation: ,
or
, where
.
Gas constant ,
.
For methane having a molar mass , the gas constant is
.
At high pressures and temperatures typical for main gas pipelines, various models of real gases are used, which has the phenomenon of supercompressibility. These models are described by the corrected Mendeleev-Cliperon equation: , where is the supercompressibility coefficient, which for real gases is always less than one;
- reduced pressure;
- reduced pressure.
There are various empirical formulas for calculating the supercompressibility factor, such as.
For a mixture of gases, the critical pressure is determined by the following formula: , and the critical temperature is found as follows:
.
Typical parameters of natural gas components:
Component name | , ![]() | , | , ![]() | , ![]() | , |
Methane | 16.042 | 0.717 | 518.33 | 4.641 | 190.55 |
Ethane ![]() | 30.068 | 1.356 | 276.50 | 4.913 | 305.50 |
Propane ![]() | 44.094 | 2.019 | 188.60 | 4.264 | 369.80 |
Nitrogen | 28.016 | 1.251 | 296.70 | 3.396 | 126.2 |
Hydrogen sulfide | 34.900 | 1.539 | 238.20 | 8.721 | 378.56 |
Carbon dioxide | 44.011 | 1.976 | 189.00 | 7.382 | 304.19 |
Air | 28.956 | 1.293 | 287.18 | 3.180 | 132.46 |
45. Gas mixtures and calculation of their parameters. Calculation of the critical parameters of the gas mixture.
INTRODUCTION
1.1 General
1.1.1 The course project (gas supply to the village of Kinzebulatovo) was developed on the basis of the general plan of the settlement.
1.1.2 When developing a project, the requirements of the main regulatory documents are taken into account:
- updated edition of SNiP 42-01 2002 "Gas distribution networks".
- SP 42-101 2003 "General provisions for the design and construction of gas distribution systems from metal and polyethylene pipes."
- GOST R 54-960-2012 “Gas control block stations. Cabinet-type gas reduction points ”.
1.2 General information about the settlement
1.2.1 There are no industrial and public utility companies on the territory of the settlement.
1.2.2 The settlement is built up with one-storey buildings. The village does not have centralized heating and centralized hot water supply.
1.2.3 Gas distribution systems in the territory of the settlement are made underground from steel pipes. Modern gas distribution systems are a complex complex of structures, consisting of the following main elements of gas ring, dead-end and mixed networks of low, medium, high pressure, laid on the territory of a city or other settlement within blocks and inside buildings, on highways - on the highways of gas control stations (GRS).
DESCRIPTION OF THE CONSTRUCTION AREA
2.1 General information about the settlement
Kinzebulatovo, Kinzebulat(head. Kinyәbulat) - a village in the Ishimbay region of the Republic of Bashkortostan, Russia.
Administrative center of the rural settlement "Baiguzinsky village council".
The population is about 1,000 people. Kinzebulatovo is located 15 km from the nearest city - Ishimbay - and 165 km from the capital of Bashkortostan - Ufa.
Consists of two parts - a Bashkir village and a former oil industry settlement.
The Tyruk River flows.
There is also the Kinzebulatovskoye oil field.
Agribusiness - Association of Peasant Farms "Udarnik"
CALCULATION OF NATURAL GAS COMPOSITION
3.1 Features of gas fuel
3.1.1 Natural gas has a number of advantages over other fuels:
- low cost;
- high heat of combustion;
- long-distance transportation of gas through main gas pipelines;
- complete combustion facilitates the working conditions of personnel, maintenance of gas equipment and networks,
- the absence of carbon monoxide in the gas, which makes it possible to avoid poisoning in the event of a leak;
- gas supply to cities and towns significantly improves the condition of their air basin;
- the ability to automate combustion processes to achieve high efficiency;
- less emission when burning harmful substances than when burning solid or liquid fuels.
3.1.2. Natural gas fuels are composed of combustible and non-combustible components. The more combustible part of the fuel, the higher the specific heat of its combustion. The combustible part or organic mass includes organic compounds, which include carbon, hydrogen, oxygen, nitrogen, sulfur. The non-combustible part consists of the hall and moisture. The main components of natural gas are methane СН 4 from 86 to 95%, heavy hydrocarbons С m Н n (4-9%), ballast impurities are nitrogen and carbon dioxide. The methane content in natural gases reaches 98%. The gas is colorless and odorless, so it is odorized. Natural combustible gases according to GOST 5542-87 and GOST 22667-87 consist mainly of methane hydrocarbons.
3.2 Combustible gases used for gas supply. Physical properties of gas.
3.2.1 Natural artificial gases are used for gas supply in accordance with GOST 5542-87, the content of harmful impurities in 1 g / 100m 3 of gas should not exceed:
- hydrogen sulfide - 2g;
- ammonia - 2g;
- cyanide compounds - 5;
- resin and dust - 0.1 g;
- naphthalene - 10g. summer and 5y. in winter.
- gases from purely gas fields. They consist mainly of methane, are dry or lean (no more than 50 g / m 3 propane and above);
- associated gases of oil fields, contain a large amount of hydrocarbons, usually 150 g / m 3, are fat gases, it is a mixture of dry gas, propane-butane fraction and gasoline.
- gases of condensate fields, it is a mixture of dry gas and condensate. Condensate vapors are a mixture of heavy hydrocarbon vapors (gasoline, naphtha, kerosene).
3.2.3. Calorific value of gas, purely gas fields, from 31000 to 38000 kJ / m 3, and associated gases of oil fields from 38000 to 63000 kJ / m 3.
3.3 Calculation of the composition of natural gas from the Proletarskoye field
Table 1-Gas composition of the Proletarskoye field
3.3.1 Net calorific value and density of natural gas components.
3.3.2 Calculation of the calorific value of natural gas:
0.01 (35.84 * CH 4 + 63.37 * C 2 H 6 + 93.37 * C 3 H 8 + 123.77 * C 4 H 10 + 146.37 * C 5 H 12), (1 )
0.01 * (35.84 * 86.7+ 63.37 * 5.3+ 93.37 * 2.4 + 123.77 * 2.0+ 146.37 * 1.5) = 41.34 MJ / m 3.
3.3.3 Determination of the density of gas fuel:
Gas = 0.01 (0.72 * CH 4 + 1.35 * C 2 H 6 + 2.02 * C 3 H 8 + 2.7 * C 4 H 10 + 3.2 * C 5 H 12 +1.997 * C0 2 + 1.25 * N 2); (2)
Gas = 0.01 * (0.72 * 86.7 + 1.35 * 5.3 + 2.02 * 2.4 + 2.7 * 2.0 + 3.2 * 1.5 + 1.997 * 0 , 6 +1.25 * 1.5) = 1.08 kg / N 3
3.3.4 Determination of the relative density of gaseous fuel:
where air is 1.21-1.35 kg / m 3;
ρ rel , (3)
3.3.5 Determination of the amount of air required for combustion of 1 m 3 of gas theoretically:
[(0.5CO + 0.5H 2 + 1.5H 2 S + ∑ (m +) C m H n) - 0 2]; (4)
V = ((1 +) 86.7 + (2 +) 5.3 + (3 +) 2.4 + (4 +) 2.0 + (5 +) 1.5 = 10.9 m 3 / m 3;
V = = 1.05 * 10.9 = 11.45 m 3 / m 3.
3.3.6 The characteristics of gaseous fuel determined by the calculation are summarized in Table 2.
Table 2 - Gas fuel characteristics
Q MJ / m 3 | R gas kg / N 3 | R rel. kg / m 3 | V m 3 / m 3 | V m 3 / m 3 |
41,34 | 1,08 | 0,89 | 10,9 | 11,45 |
GAS PIPELINE ROUTING
4.1 Classification of gas pipelines
4.1.1 Gas pipelines laid in cities and towns are classified according to the following indicators:
–By the type of transported natural gas, associated gas, petroleum gas, liquefied hydrocarbon gas, artificial gas, mixed gas;
- by gas pressure of low, medium and high (I category and II category); –For the deposit relative to the ground: underground (underwater), aboveground (above water);
- by location in the planning system of cities and settlements, external and internal;
–On the principle of construction (distribution gas pipelines): loopback, dead-end, mixed;
- according to the pipe material, metal, non-metal.
4.2 Selection of the gas pipeline route
4.2.1 The gas distribution system can be reliable and economical with the right choice of routes for laying gas pipelines. The choice of the route is influenced by the following conditions: distance to gas consumers, direction and width of passages, type of road surface, presence of various structures and obstacles along the route, terrain, layout
quarters. Gas pipelines are selected taking into account the transportation of gas by the shortest route.
4.2.2 From street gas pipelines, inlets are laid into each building. In urban areas with a new layout, gas pipelines are located within blocks. When routing gas pipelines, it is necessary to observe the distance of gas pipelines from other structures. It is allowed to lay two or more gas pipelines in one trench at the same or different levels (steps). In this case, the distance between gas pipelines in the light should be sufficient for the installation and repair of pipelines.
4.3 Basic provisions for laying gas pipelines
4.3.1 Laying of gas pipelines should be carried out at a depth of at least 0.8 m to the top of the gas pipeline or case. In places where the movement of vehicles and agricultural machinery is not foreseen, the depth of laying steel gas pipelines is allowed at least 0.6 m.On landslide and erosion-prone areas, laying gas pipelines should be provided for a depth of at least 0.5 m below the sliding surface and below the predicted boundary. area of destruction. In justified cases, it is allowed to lay gas pipelines on the ground along the walls of buildings inside residential yards and quarters, as well as on bleaching sections of the route, including at sections of crossings through artificial and natural barriers when crossing underground utilities.
4.3.2 Aboveground and aboveground gas pipelines with embankment can be laid in rocky, permafrost soils, in swampy areas and in other difficult soil conditions. The material and dimensions of the embankment should be taken on the basis of the heat engineering calculation, as well as ensuring the stability of the gas pipeline and the embankment.
4.3.3 Laying gas pipelines in tunnels, collectors and channels is not allowed. Exceptions are the laying of steel gas pipelines with a pressure of up to 0.6 MPa on the territory of industrial enterprises, as well as in channels of permafrost soils under roads and railways.
4.3.4 Pipe connections should be provided as one-piece. Connections of steel pipes with polyethylene pipes can be detachable and in places where fittings, equipment and instrumentation are installed. Detachable joints of polyethylene pipes with steel pipes in the ground can only be provided if a case with a control tube is installed.
4.3.5 Gas pipelines at the points of entry and exit from the ground, as well as gas pipelines inlets to buildings should be enclosed in a case. In the space between the wall and the case, the entire thickness of the structure to be crossed should be sealed. The ends of the case should be sealed with an elastic material. Entries of gas pipelines into buildings should be provided directly for the room where the gas-using equipment is installed, or adjacent rooms, connected by a covered opening. It is not allowed to enter gas pipelines into the premises of the basement and basement floors of buildings, except for the entries of natural gas pipelines into single-family and blocked houses.
4.3.6 The disconnecting device on gas pipelines should be provided for:
- in front of detached blocked buildings;
- to disconnect risers of residential buildings above five floors;
- in front of outdoor gas-using equipment;
- in front of gas control points, with the exception of the hydraulic fracturing station of the enterprise, at the branch of the gas pipeline to which there is a disconnecting device at a distance of less than 100 m from the hydraulic fracturing station;
- at the exit from gas control points, looped gas pipelines;
- on branches from gas pipelines to settlements, separate microdistricts, quarters, groups of residential buildings, and with more than 400 apartments, to a separate house, as well as on branches to industrial consumers and boiler houses;
- when crossing water barriers with two or more lines, as well as one line with a water barrier width with a low water level of 75 m or more;
- at the intersection of railways of the general network and highways of 1–2 categories, if a disconnecting device that ensures the cessation of gas supply at the crossing section located at a distance of more than 1000 m from the roads.
4.3.7 Disconnecting devices on aboveground gas pipelines,
laid along the walls of buildings and on supports, should be placed at a distance (within a radius) from door and opening window openings at least:
- for low pressure gas pipelines - 0.5 m;
- for gas pipelines of medium pressure - 1 m;
- for high-pressure gas pipelines of the second category - 3 m;
- for high-pressure gas pipelines of the first category - 5 m.
Installation of disconnecting devices on the sections of transit laying of gas pipelines along the walls of buildings is not allowed.
4.3.8 The vertical distance (in the light) between the gas pipeline (case) and underground utilities and structures at their intersection should be taken taking into account the requirements of the relevant regulatory documents, but not less than 0.2 m.
4.3.9 At the intersection of gas pipelines with underground utilities, collectors and channels for various purposes, as well as at the places where gas pipelines pass through the walls of gas wells, the gas pipeline should be laid in a case. The ends of the case should be brought out at a distance of at least 2 m in both directions from the outer walls of the structures and communications being crossed, when crossing the walls of gas wells - at a distance of at least 2 cm. The ends of the case should be sealed with waterproofing material. At one end of the case, at the upper points of the slope (with the exception of the intersections of the walls of the wells), a control tube should be provided that goes under the protective device. In the annular space of the case and the gas pipeline, it is allowed to lay a service cable (communication, telemechanics and electrical protection) with a voltage of up to 60V, intended for servicing gas distribution systems.
4.3.10 Polyethylene pipes used for the construction of gas pipelines must have a safety factor for GOST R 50838 of at least 2.5.
4.3.11 Laying of gas pipelines from polyethylene pipes is not allowed:
- on the territory of settlements at a pressure of over 0.3 MPa;
- outside the territory of settlements at a pressure of over 0.6 MPa;
- for transportation of gases containing aromatic and chlorinated hydrocarbons, as well as the liquid phase of LPG;
- at a gas pipeline wall temperature under operating conditions below –15 ° С.
When using pipes with a safety factor of at least 2.8, it is allowed to lay polyethylene gas pipelines with a pressure of more than 0.3 to 0.6 MPa in the territories of a settlement with mainly one-two-story and cottage residential buildings. On the territory of small rural settlements, it is allowed to lay polyethylene gas pipelines with a pressure of up to 0.6 MPa with a safety factor of at least 2.5. In this case, the laying depth should be at least 0.8 m to the top of the pipe.
4.3.12 The strength analysis of gas pipelines should include the determination of the wall thickness of pipes and fittings and the stresses in them. At the same time, pipes and fittings with a wall thickness of at least 3 mm should be used for underground and above-ground steel gas pipelines, and at least 2 mm for above-ground and internal gas pipelines.
4.3.13 Characteristics of limiting states, reliability factors for liability, standard and calculated values of loads and effects and their combinations, as well as standard and calculated values of characteristics of materials should be taken in calculations taking into account the requirements of GOST 27751.
4.3.14 During construction in areas with difficult geological conditions and seismic effects, special requirements must be taken into account and measures must be taken to ensure the strength, stability and tightness of gas pipelines. Steel gas pipelines must be protected against corrosion.
4.3.15 Underground and surface steel pipelines with embankments, LPG tanks, steel inserts for polyethylene gas pipelines and steel cases on gas pipelines (hereinafter referred to as gas pipelines) should be protected against soil corrosion and corrosion by stray currents in accordance with the requirements of GOST 9.602.
4.3.16 Steel cases of gas pipelines under roads, railways and tramways during trenchless laying (puncture, punching and other technologies permitted for use) must, as a rule, be protected by means of electrical protection (3X3), when laid in an open way - by insulating coatings and 3X3.
4.4 Selection of material for the gas pipeline
4.4.1 For underground gas pipelines, polyethylene and steel pipes should be used. For aboveground and aboveground gas pipelines, steel pipes should be used. For internal low pressure gas pipelines, it is allowed to use steel and copper pipes.
4.4.2 Steel seamless, welded (longitudinal and spiral seam) pipes and fittings for gas distribution systems should be made of steel containing not more than 0.25% carbon, 0.056% sulfur and 0.04% phosphorus.
4.4.3 The choice of material for pipes, pipeline valves, fittings, welding materials, fasteners and others should be made taking into account the gas pressure, the diameter and thickness of the gas pipeline wall, the design temperature of the outside air in the construction area and the temperature of the pipe wall during operation, ground and natural conditions, the presence of vibration loads.
4.5 Overcoming natural obstacles with a gas pipeline
4.5.1 Overcoming natural obstacles by gas pipelines. Natural obstacles are water barriers, ravines, gorges, gullies. Gas pipelines at underwater crossings should be laid with deepening into the bottom of the crossed water barriers. If necessary, according to the results of calculations for ascent, it is necessary to ballast the pipeline. The elevation of the top of the gas pipeline (ballast, lining) should be at least 0.5 m, and at crossings through navigable and floatable rivers - 1.0 m lower than the predicted bottom profile for a period of 25 years. When performing works by the method of directional drilling - not less than 20 m below the predicted bottom profile.
4.5.2 At underwater crossings the following should be applied:
- steel pipes with a wall thickness of 2 mm more than the calculated one, but not less than 5 mm;
- polyethylene pipes having a standard dimensional ratio of the outer diameter of the pipe to the wall thickness (SDR) of no more than 11 (according to GOST R 50838) with a safety factor of at least 2.5.
4.5.3 The height of the laying of the above-water passage of the gas pipeline from the design level of the rise of water or ice drift (high water horizon - GVV or ice drift - GVL) to the bottom of the pipe or superstructure should be taken:
- at the intersection of ravines and beams - not less than 0.5 m and above the GVV 5% coverage;
- when crossing non-navigable and non-floating rivers - not less than 0.2 m above the GWV and GVL of 2% supply, and if there is a grubber on the rivers - taking it into account, but not less than 1 m above the GWV of 1% supply;
- when crossing navigable and floating rivers - not less than the values established by the design standards for bridge crossings on navigable rivers.
4.5.4 Stop valves should be placed at a distance of at least 10 m from the boundaries of the crossing. The places where the gas pipeline crosses the high water horizon with 10% coverage are taken as the border of the crossing.
4.6 Crossing artificial obstacles with a gas pipeline
4.6.1 Crossing artificial obstacles by gas pipelines. Artificial obstacles are highways, railways and tramways, as well as various embankments.
4.6.2 The horizontal distance from the intersection of underground gas pipelines tram and railway tracks and highways must be at least:
- to bridges and tunnels on public railways, tramways, highways of 1 - 3 categories, as well as to pedestrian bridges, tunnels through them - 30 m, and for non-public railways, highways of 4 - 5 categories and pipes - 15m;
- to the zone of the turnout transport (the beginning of the wits, the tail of the crosspieces, the points of connection to the rails of the suction cables and other crossings of the track) - 4m for tram tracks and 20m for the railways;
- up to the overhead supports - 3m.
4.6.3 It is allowed to reduce the specified distances in agreement with the organizations in charge of the crossed structures.
4.6.4 Underground gas pipelines of all pressures at intersections with railway and tram tracks, highways of categories 1 - 4, as well as main streets of city-wide importance should be laid in cases. In other cases, the question of the need for the device of cases is decided by the design organization.
4.7 Cases
4.7.1 Cases must meet the conditions of strength and durability. A test tube should be provided at one end of the case, which extends under the protective device.
4.7.2 When laying inter-settlement gas pipelines in cramped conditions and gas pipelines on the territory of settlements, it is allowed to reduce this distance to 10 m, provided that an exhaust plug with a sampling device is installed at one end of the case, brought out at a distance of at least 50 m from the edge of the roadbed (the axis of the extreme rail at zero marks). In other cases, the ends of the cases should be spaced at a distance:
- not less than 2 m from the end rail of the tramway and railways, potassium 750 mm, as well as from the edge of the carriageway of streets;
- not less than 3m from the edge of the drainage structure of roads (ditch, ditch, reserve) and from the extreme rail of non-public railways, but not less than 2m from the foot of the embankments.
4.7.3 The depth of laying the gas pipeline from the foot of the rail or the top of the road surface, and in the presence of an embankment, from its bottom to the top of the case, must meet safety requirements, not less than:
- when performing work by an open method - 1.0 m;
- when performing works by punching shear or directional drilling and shield laying - 1.5 m;
- when performing work by the puncture method - 2.5 m.
4.8. Crossing pipes with roads
4.8.1 The thickness of the walls of the pipes of a steel gas pipeline when it crosses public railways should be 2 - 3 mm more than the calculated one, but not less than 5 mm at distances of 50 m in each direction from the edge of the roadbed (the axis of the outer rail at zero marks).
4.8.2 For polyethylene gas pipelines in these sections and at the intersections of highways of categories 1 - 3, polyethylene pipes of no more than SDR 11 with a safety factor of at least 2.8 should be used.
4.9 Corrosion protection of pipelines
4.9.1 Pipelines used in gas supply systems are usually made of carbon and low-alloy steels. The service life and reliability of pipelines are largely determined by the degree of protection against destruction upon contact with the environment.
4.9.2 Corrosion is the destruction of metals caused by chemical or electrochemical processes when interacting with the environment. The environment in which metal corrodes is called corrosive or corrosive.
4.9.3 The most relevant for underground pipelines is electrochemical corrosion, which obeys the laws of electrochemical kinetics, this is the oxidation of metal in conductive media, accompanied by the formation and flow of electric current. In this case, the interaction with the environment is characterized by cathodic and anodic processes occurring in different areas of the metal surface.
4.9.4 All underground steel pipelines laid directly into the ground are protected in accordance with GOST 9.602-2005.
4.9.5 In soils of medium corrosive activity in the absence of stray currents, steel pipelines are protected by insulating coatings of "very reinforced type", in soils of high corrosiveness of the dangerous influence of stray currents - by protective coatings of "very reinforced type" with the obligatory use of 3X3.
4.9.6 All envisaged types of protection against corrosion are put into operation the distribution of underground pipelines into operation. For underground steel pipelines in zones of dangerous influence of stray currents, 3X3 is put into operation no later than 1 month, and in other cases later than 6 months after laying the pipeline in the ground.
4.9.7 The corrosive aggressiveness of soil in relation to steel is characterized in three ways:
- the specific electrical resistance of the soil, determined in the field;
- specific electrical resistance of soil, determined in laboratory conditions,
- the average density of the cathodic current (j k) required to displace the potential of steel in the soil by 100 mV more negative than the stationary one (corrosion potential).
4.9.8 If one of the indicators indicates a high aggressiveness of the soil, then the soil is considered aggressive, and the determination of the remaining indicators is not required.
4.9.9 The dangerous influence of a stray direct current on underground steel pipelines is the presence of a changing in sign and in magnitude of the displacement of the pipeline potential in relation to its stationary potential (sign-alternating zone) or the presence of only a positive displacement of the potential, which, as a rule, varies in magnitude (anode zone) ... For the designed pipelines, the presence of stray currents in the ground is read as dangerous.
4.9.10 The dangerous effect of alternating current on steel pipelines is characterized by a displacement of the average potential of the pipeline in the negative direction by at least 10 mV, in relation to the stationary potential, or the presence of alternating current with a density of more than 1 MA / cm 2. (10 A / m 2.) On the auxiliary electrode.
4.9.11 The use of 3X3 is mandatory:
- when laying pipelines in soils with high corrosive aggressiveness (protection against soil corrosion),
- in the presence of a dangerous influence of constant stray and alternating currents.
4.9.12 When protecting against soil corrosion, cathodic polarization of underground steel pipelines is carried out in such a way that the average value of the polarization potentials of the metal is within the range of –0.85V. up to 1.15V on a saturated copper-sulfate electrode in comparison (m.s.e.).
4.9.13 Insulation work in route conditions is performed manually when insulating prefabricated joints and small fittings, correcting damage to the coating (no more than 10% of the pipe area) that occurred during pipe transportation, as well as during pipeline repair.
4.9.14 When repairing damage to the factory insulation on site, laying the gas pipeline, it must be ensured that the technology and technical capabilities of coating and control of its quality are observed. All works on the repair of the insulating coating are reflected in the passport of the gas pipeline.
4.9.15 Polyethylene, polyethylene tapes, bitumen and bitumen-polymer mastics, fused bitumen-polymer materials, roll mastic-tape materials, compositions based on chlorosulfonated polyethylene, polyester resins and polyurethanes are recommended as the main materials for the formation of protective coatings.
DETERMINATION OF GAS FLOWS
5.1 Gas consumption
5.1.1 Gas consumption by network sections can be conditionally divided into:
track, transit and dispersed.
5.1.2 Path flow rate is a flow rate that is evenly distributed along the length of a section or the entire gas pipeline, equal or very close in value. It can be selected through the same size and for ease of calculation, it is evenly distributed. Usually, this consumption is consumed by gas appliances of the same type, for example, capacitive or instantaneous water heaters, gas stoves, etc. Lumped flow rates are those that pass through the pipeline, without changing, along the entire length and are sampled at certain points. The consumers of these costs are: industrial enterprises, boiler houses with a constant consumption for a long time. Transit costs are those that pass through a certain section of the network, without changing, and provide gas consumption, to the next section, being for it track or concentrated.
5.1.2 Gas consumption in the settlement is travel or transit. There are no concentrated gas expenditures, since there are no industrial enterprises. Travel costs are made up of the costs of gas appliances installed by consumers, and depends on the season of the year. The apartment has four Glem UN6613RX burner plates with a gas flow rate of 1.2 m 3 / h, a Vaillant instantaneous water heater for hot flow with a flow rate of 2 m 3 / h, Viessmann Vitocell-V 100 CVA- 300 "with a flow rate of 2.2 m 3 / h.
5.2 Gas consumption
5.2.1 Gas consumption changes by hours, days, days of the week, months of the year. Depending on the t period during which, gas consumption is taken to be constant, they are distinguished: seasonal irregularity or irregularity in the months of the year, daily irregularity or irregularity in the days of the week, hourly irregularity or irregularity in the hours of the day.
5.2.2 Unevenness of gas consumption is associated with seasonal climatic changes, operating mode of enterprises during the season, week and day, characteristics of gas equipment of various consumers, studies of unevenness are built stepwise gas consumption over time. To regulate the seasonal unevenness of gas consumption, the following methods are used:
- underground gas storage;
- the use of consumers of regulators that dump surpluses in the summer;
- reserve fields and gas pipelines.
5.2.3 To regulate the unevenness of gas consumption of gas in the winter months, gas is taken from underground storage facilities, and in a short period of the year, it is pumped into underground storage facilities. To cover daily peak loads, the use of underground storage facilities is not economical. In this case, restrictions on gas supply to industrial enterprises are introduced and peak coverage stations are used, in which gas liquefaction occurs.
For the gas composition, determined from the average component composition of natural gas, depending on the field, it is necessary to calculate the characteristics of the gaseous fuel. The characteristics of natural gas are given in table 1.
Table 1 - Composition of gas by volume for various fields
Gas component |
CH 4 |
WITH 2 H 6 |
WITH 3 H 8 |
WITH 4 H 10 |
WITH 5 H 12 |
N 2 |
CO 2 |
H 2 S |
Field |
Severostavropol'skoe, Stavropol Territory |
|||||||
Field |
Medvezhye, Tyumen region |
|||||||
Field |
Vaneivskoe, Arkhangelsk region |
|||||||
Field |
Zapolyarnoe, Tyumen region |
|||||||
Field |
Layavozh, Arkhangelsk region |
|||||||
Field |
Vasilkovskoe, Arkhangelsk region |
|||||||
Gas calorific value- the amount of heat that can be obtained with the complete combustion of 1 m3 of gas under normal conditions.
Distinguish between higher and lower calorific value of fuel.
Gross calorific value of gas- the amount of heat obtained during the complete combustion of 1m3 of gas, including the heat released during the condensation of water vapor of the combustion products.
Net calorific value of gas- the amount of heat received in the combustion process, excluding the heat of condensation of water vapor - combustion products.
In practice, when gas is burned, water vapor does not condense, but is removed with other combustion products, therefore, the calculation is based on the lower calorific value of the gas.
The heat of combustion (highest or lowest) of dry gaseous fuel (gas) is determined by the formula
,
(1)
where Q c - heat of combustion of dry gas, kJ / m 3;
Q 1, Q 2, Q k is the heat of combustion of the components that make up the gaseous fuel, kJ / m 3;
x 1, x 2, x 3 - volume fractions of components making up gaseous fuel,%.
Table 2 - Heat of combustion of pure combustible gases
Heat of combustion |
||
|
||
Isobutane | ||
Carbon monoxide | ||
Hydrogen sulfide |
The density of dry gas is determined as the sum of the products of the densities of the components that make up the gaseous fuel by their volume fractions:
,
(2)
where p is the density of dry gas, kg / m 3;
p 1, p 2,…, p k - component densities, kg / m 3.
Table 3 - Physical characteristics of gases
Gas composition |
Density. kg / m 3 att = 0 0 C P =101.3 kPa |
Relative density by air |
Methane CH 4 | ||
Ethane C 2 H 6 | ||
Propane C 3 H 8 | ||
Butane C 4 H 10 | ||
Isobutane C 5 H 12 | ||
Carbon dioxide CO 2 | ||
Hydrogen sulfide H 2 S |
The relative density of dry gas in air is:
,
(3)
where p in = 1.293 - air density under normal conditions, kg / m 3.
Gas characteristics are summarized in Table 4.
Table 4 - Characteristics of gaseous fuel under normal physical conditions (T = 273.15 K, P = 101.325 kPa)